Impact of morphology and confinement effects on the properties of aligned nanofiber architectures
Author(s)
Stein, Itai Y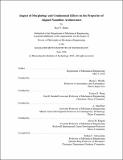
DownloadFull printable version (9.321Mb)
Other Contributors
Massachusetts Institute of Technology. Department of Mechanical Engineering.
Advisor
Brian L. Wardle.
Terms of use
Metadata
Show full item recordAbstract
The intrinsic and scale-dependent properties of nanofibers (NFs), nanowires, and nanotubes have made them the focus of many application-specific nanostructured materials studies. However, various NF morphology and proximity effects can lead to > 1000x reductions in the performance of NF-based material architectures, such as bulk materials and structures comprised of scalable aligned NF arrays. The physical and chemical origins of these effects, along with the concomitant structure property mechanisms of materials comprised of aligned NFs, are not currently known and cannot be properly integrated into existing theories. This originates, in part, from an incomplete understanding of the morphology of real NF systems, particularly in three-dimensions. Through experiments, theory, and multi-scale simulation, this dissertation presents a framework capable of modeling the stochastic 3D morphology of a relevant NF system, carbon nanotubes (CNTs), assembled into aligned CNT (A-CNT) arrays. New descriptions of the multi-wall A-CNT morphology demonstrate that the CNT tortuosity, quantified via sinusoidal amplitude-wavelength waviness ratio (w), decreases significantly from w ~/~ 0:2 to 0:1 as the CNT volume fraction (Vf) is increased from Vf ~ 1 to 20%. Using these new relations, a 3D stochastic morphology description is presented, and used to quantify the mechanical behavior of A-CNT arrays, A-CNT polymer matrix nanocomposites (A-PNCs), and A-CNT carbon matrix nanocomposites (A-CMNCs) via a mechanics analysis that was previously applied to carbon nanocoils. Focusing on deformations in the A-CNT axial reinforcement direction, torsion and shear deformation mechanisms, which are governed by the low (< 1 GPa) intrinsic shear modulus of the CNTs, are shown to have an effective compliance contribution of > 90% in the experimental A-CNT w regime, and are inferred to be the physical mechanisms responsible for the previously observed ~ 100x increase in the ACNT effective indentation modulus as Vf is increased from ~ 1 to 20%. In the case of A-PNCs, the polymer matrix effectively eliminates the torsion compliance contribution, so that the observed ~ 2x enhancement in the effective axial elastic modulus of A-PNCs as Vf is increased from ~ 1 to 20% is explained. The geometry of the graphitic crystallites that comprise the pyrolytic carbon (PyC) matrix of A-CMNCs is found to not evolve significantly at pyrolysis temperatures of 1000 to 1400°C, and crystallite size estimates from Raman spectroscopy reveal that the Tuinstra-Koenig correlation disagrees with the sizes measured by x-ray diffraction, suggesting a new amorphization transition crystallite size of 6 nm instead of 2-3 nm. In the case of A-CMNCs, CNT reinforcement is shown to lower the energy barrier (inferred through the pyrolysis temperature) for meso-scale self-organization of the graphitic crystallites of the PyC matrix, while having no effect on the PyC matrix on the atomic scale. Mechanical property analysis and modeling indicates that the aerospace materials selection criterion of the A-CMNCs can be enhanced to >8 GPa x (g/cm3)-2 at Vf >20% (experimentally we observe a value of ~ 5 GPa x (g/cm3)-2 at Vf ~ 10%). A-CMNCs introduced in this work have the potential to outperform state-of-the-art superhard materials, such as diamond (~/~ 7:8 GPa x (g/cm3)-2) and cubic boron nitride (~/~ 5:2 GPa x (g/cm3)-2). Using the structure-property prediction tools developed in this thesis, precise tailoring and prediction of application-specific performance of aligned NF based architectures is enabled, and specific new understanding of A-CNT systems is established. Future paths of study that enable the design and manufacture of several classes of next-generation materials are recommended.
Description
Thesis: Ph. D., Massachusetts Institute of Technology, Department of Mechanical Engineering, 2016. This electronic version was submitted by the student author. The certified thesis is available in the Institute Archives and Special Collections. Cataloged from student-submitted PDF version of thesis. Includes bibliographical references (pages 151-168).
Date issued
2016Department
Massachusetts Institute of Technology. Department of Mechanical EngineeringPublisher
Massachusetts Institute of Technology
Keywords
Mechanical Engineering.