Designing devices using electro-active yield stress fluids
Author(s)
Helal, Ahmed H., Ph. D. Massachusetts Institute of Technology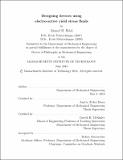
DownloadFull printable version (17.14Mb)
Alternative title
Designing devices using EAYS
Other Contributors
Massachusetts Institute of Technology. Department of Mechanical Engineering.
Advisor
Anette (Peko) Hosoi and Gareth H. McKinley.
Terms of use
Metadata
Show full item recordAbstract
Understanding the rheo-electrical properties of electro-active yield stress fluids (EAYS) lies at the heart of enabling the design of a number of novel applications such as smart valves and semi-solid flow batteries. This thesis explores the rheological and electrical properties of EAYS fluids, with a focus on how the presence of a deformable microstructure, which gives rise to a yield stress at the macroscale, affects the design and performance of the corresponding devices. This thesis will focus on two representative case studies of such devices using EAYS fluids: 1) miniaturized, rapidly-actuated hydraulic valves using electro-rheological fluids applied to centimeter-scale robotics and 2) semi-solid flow batteries using conductive suspensions of percolated carbon black networks flowing in millifluidic channels. Through experimental characterization and modeling of the rheo-electric properties of the materials, this thesis investigates the interplay between the electrical and rheological properties of the EAYS fluids used in these devices. We demonstrate how an improved understanding of the flow of EAYS fluids and the development of device-level predictive models of the key performance metrics leads to a better understanding of the device design and operation space. In the first part of this thesis, we characterize the field-dependent yield stress of electrorheological fluids at different electric fields and particle volume fractions in wall-driven and pressure-driven flow. We report a yield hardening occurring in pressure-driven flow, as a result of a local increase in particle volume fraction. We propose a phenomenological physical model for the densification process, allowing us to reconcile rheological measurements from wall-driven shear flows e.g. torsional rheometers and in pressure-driven channel flows, that are more applicable to valve applications. Next, we extend this work by introducing the holding pressure drop and power efficiency as key performance metrics for electrorheological valves. Using a custom modular valve design, we investigate the effects of the aspect ratio of the valve and electrode surface roughness on the valve performance. This study enables us to derive design rules for ER valve design and to demonstrate their applicability in robotic applications. In the second part of this thesis, we characterize the rheological and electrical properties of carbon black gels under steady and oscillatory shear flow. We assess the influence of design parameters such as the carbon loading, surface material and choice of additives on the rheology and wall-slip behavior of lithium polysuflide semi-solid suspensions. We discuss the design of a new rheo-electric test fixture for controlled stress rheometers; enabling simultaneous measurements of the rheo-electric properties of strongly conductive materials such as semi-solid flow battery slurries. After careful calibration of the new test fixture, we demonstrate its relevance as a versatile characterization tool by studying the rheo-electric properties of a series of model carbon black gels at rest and under transient and steady shear. Additionally, we show that small amplitude oscillatory shear measurements, performed concomitantly to conductivity measurements on carbon black gels, allow us to precisely extrapolate the conductivity and rigidity percolation thresholds of the carbon black gels. Large amplitude oscillatory shear measurements are also shown to be a useful method to study fatigue in carbon black gels at stresses close to the yield stress. Finally, we introduce a new pumpless design architecture for flow cells: the Gravity-Induced Flow Cell (GIFcell), that is driven by gravity. We demonstrate how insight into the rheological and wall slip behavior of the lithium polysulfide semi-solid suspensions obtained through careful characterization informs and helps optimize cell design and operation.
Description
Thesis: Ph. D., Massachusetts Institute of Technology, Department of Mechanical Engineering, 2016. This electronic version was submitted by the student author. The certified thesis is available in the Institute Archives and Special Collections. Cataloged from student-submitted PDF version of thesis. Includes bibliographical references (pages 199-223).
Date issued
2016Department
Massachusetts Institute of Technology. Department of Mechanical EngineeringPublisher
Massachusetts Institute of Technology
Keywords
Mechanical Engineering.