Novel angular and frequency manipulation of light in nano-scaled dielectric photonic systems
Author(s)
Shen, Yichen, Ph. D. Massachusetts Institute of Technology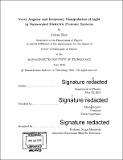
DownloadFull printable version (12.18Mb)
Other Contributors
Massachusetts Institute of Technology. Department of Physics.
Advisor
Marin Soljačić.
Terms of use
Metadata
Show full item recordAbstract
Humankind has long endeavored to control light. In modern society, with the rapid development of nanotechnology, the control of light is moving toward devices at micrometer and even nanometer scales. At such scales, traditional devices based on geometrical optics reach their fundamental diffraction limits and cease to work. Nano-photonics, on the other hand, has attracted wide attention from researchers, especially in the last decade, due to its ability to manipulate light at the nanoscale. In this thesis, we explore novel control of light created by nanophotonic structures, with a common theme on light interference in nanoscaled dielectric photonic systems. The first part of the thesis focuses on broadband angular selective nanophotonic systems. We survey the literatures and the current state of the art focused on enabling optical broadband angular selectivity. We also present a novel way of achieving broadband angular selectivity using Brewster mode in nanophotonic systems. We propose two categories of potential applications for broadband angularly selective systems. The first category aims at enhancing the efficiency of solar energy harvesting, through photovoltaic process or solar thermal process. The second category aims at enhancing light extracting efficiency and detection sensitivity. Finally, we discuss the most prominent challenges in broadband angular selectivity and some prospects on how to solve these challenges. The second part of the thesis focuses on spectrum control of light using all-dielectric surface resonator. We proposes a new structural color generation mechanism that produces colors by the Fano resonance effect on thin photonic crystal slab. We experimentally realize the proposed idea by fabricating the samples that show resonance-induced colors with weak dependence on the viewing angle. We also show that the colors can be dynamically tuned by stretching the photonic crystal slab fabricated on an elastic substrate. In a follow up work, we address how to overcome the challenge of mode leaking on dielectric substrate. We present a class of low-index zigzag surface structure that supports resonance modes even without index contrast with the substrate. In the third part, we investigate neuromorphic computation using the interference of light in on-chip dielectric photonic waveguide network. We first mathematically prove that conventional neural networks architecture can be equivalently represented by nanoscaled optical systems. We then experimentally demonstrate that our optical neural networks are able to give equivalent accuracy on a standard training datasets. In the last part, we show that in principle optical neural nets are at least 3 orders of magnitude faster and power efficient in forward propagation than conventional neural nets.
Description
Thesis: Ph. D., Massachusetts Institute of Technology, Department of Physics, 2016. Cataloged from PDF version of thesis. Includes bibliographical references (pages 93-114).
Date issued
2016Department
Massachusetts Institute of Technology. Department of PhysicsPublisher
Massachusetts Institute of Technology
Keywords
Physics.