A methodology based on local resolution of turbulent structures for effective modeling of unsteady flows
Author(s)
Lenci, Giancarlo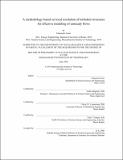
DownloadFull printable version (37.36Mb)
Other Contributors
Massachusetts Institute of Technology. Department of Nuclear Science and Engineering.
Advisor
Emilio Baglietto and Pierre F.J. Lermusiaux.
Terms of use
Metadata
Show full item recordAbstract
Computational fluid dynamics (CFD) is widely adopted to predict complex flows and to inform the design of advanced engineering systems. In particular, the industry has seen a continuous growth of CFD simulations of turbulent phenomena, which has been made possible by the tremendous reduction achieved in cost per floating point operation. The need for accurate, yet computationally effective, transient methods has drawn particular attention to hybrid turbulence models, seeking to offer significant cost reduction over large-eddy simulation (LES). Despite promising results in academic test cases, the use of hybrid modeling is still scarce in general industrial simulations. Such a low adoption is mainly driven by limitations in the ability to provide accurate, robust, reliable, and grid-convergent solutions. Hybrid models are still far from presenting a viable replacement to unsteady Reynolds-averaged Navier-Stokes (URANS). This work investigates the key weaknesses of current hybrid approaches and develops a novel modeling strategy to overcome selected shortcomings. Hybrid approaches have mostly focused on identifying regions of the flow where LES could be recovered, based for example on a comparison between grid and flow length scales. Conversely, the new approach aims at leveraging the robustness of URANS in suitable flow regions while introducing controlled eddy resolution in regions of poor URANS applicability. This drastic change in strategy focuses on avoiding the frequent failures observed for existing hybrid methods while still enabling the effective resolution of scales. The formulation proposed identifies critical flow regions based on the absolute value of the second invariant of the resolved velocity gradient tensor. The variable retains several useful properties, including the capability to describe scale separation, deformation, shear flow, and coherent structures (thus motivating the name STRUCT adopted for the new approach). The method is implemented into a finite volume, general-purpose CFD solver and tested in unsteady flow applications on URANS-like grids. Results demonstrate the capability of the model to select regions of effective hybrid activation based on local flow variables. In all flow cases tested, the hybrid model activates in areas of strong flow deformation producing large resolved structures in the solution and generating enhanced description of low-frequency phenomena compared to URANS. This observation confirms the powerful consistency between regions of low URANS performance and highly deformed structures, and supports the potential of the STRUCT approach in applications requiring selective spectral information such as fluid-structure interaction analyses. After a controlled evaluation of the model with fixed parameters specified before the simulation, two formulations are proposed to provide full closure: one based on a local averaging operator represented by a truncated Taylor series expansion, and a second one leveraging a Lagrangian differential operator to provide a smooth averaging. Both of those methods aim at providing viable averaged fields as needed by the STRUCT formulation while relying only on local operations for ensuring a low penalty on computational cost in parallel simulations. The former method determines the hybrid activation regions but still relies on a constant coefficient for reducing the modeled turbulent scales. On the other hand, the latter method assigns both conditions automatically. In all simulation results, significant agreement with experimental data is achieved for the first and second central moments of the velocity components. These results illustrate the potential of the STRUCT approach to provide accurate LES-like predictions with a computational cost only about 10% higher than that of URANS while orders of magnitude lower than LES. The generality of the STRUCT approach is confirmed in applied work extending its applicability to real flow cases. The controlled STRUCT approach demonstrates significant grid consistency properties while preventing strong error increase as the grid is coarsened beyond URANS convergence. The STRUCT methodology has the potential to revert to URANS in conditions of poor hybrid model applicability while future work can design LES retrieval at the fine-grid limit. Due to those robust properties and to the safe fail-to-URANS rationale, the STRUCT concept has the potential to achieve through future tests and developments a reliable enhancement over URANS.
Description
Thesis: Ph. D., Massachusetts Institute of Technology, Department of Nuclear Science and Engineering, 2016. This electronic version was submitted by the student author. The certified thesis is available in the Institute Archives and Special Collections. Cataloged from student-submitted PDF version of thesis. Includes bibliographical references (pages 164-175).
Date issued
2016Department
Massachusetts Institute of Technology. Department of Nuclear Science and EngineeringPublisher
Massachusetts Institute of Technology
Keywords
Nuclear Science and Engineering.