Unified framework to design efficient membrane distillation for brine concentration
Author(s)
Swaminathan, Jaichander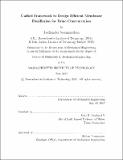
DownloadFull printable version (7.590Mb)
Other Contributors
Massachusetts Institute of Technology. Department of Mechanical Engineering.
Advisor
John H. Lienhard, V.
Terms of use
Metadata
Show full item recordAbstract
Increasing regulations requiring industrial zero-liquid discharge necessitate the development of efficient desalination technologies for treating high salinity brine. Membrane distillation (MD) is promising for such applications since it is a scalable process that that can be coupled with a low temperature heat source to concentrate water up to saturation. More than five configurations of MD, based on different condensation and heat recovery approaches, have simultaneously been under development in the literature. In this thesis, we establish a common framework to characterize the thermodynamic performance of MD systems and to rank these configurations in terms of a combination of their energy efficiency (represented as GOR) and pure water productivity (flux). A simplified heat exchanger (HX) analogy model is developed for single-stage balanced counterflow MD, based on the effectiveness-NTU method for heat exchanger analysis. This model can predict GOR and flux as a function of inlet temperatures, system size and flow rates, without using iterative finite difference solvers. A hydrophobic microporous membrane establishes the interface for evaporation in MD. In contrast to a simple heat exchanger, both heat conduction and vapor transfer occur through this membrane. While vapor transport results in pure water production, heat conduction is a loss mechanism. To account for these additional effects, two new dimensionless parameters are defined. These parameters together with NTU and feed salinity can completely characterize a general MD system's energy efficiency. Contrary to some prior design suggestions, we show that low conductivity is preferable only between the evaporation and condensation interfaces, to limit heat conduction losses. In all other regions, a high conductance is ideal, and results in improvements in both GOR and flux. Based on the above findings, a conductive gap MD (CGMD) configuration is proposed. CGMD approaches the upper-limit performance of direct contact MD and permeate gap MD, for a given membrane and channel heat transfer characteristics. Another widely used MD configuration is air gap MD (AGMD) which includes a region of stagnant air between the membrane and condensation surface. AGMD performance can also be predicted using the proposed analytical framework by considering the membrane and the air region together as the effective membrane. Since this effective membrane is thicker, less conductive and has a higher permeability coefficient, AGMD is best suited for high salinity applications. However, if the air gap region gets flooded with pure water during operation, performance can deteriorate towards the lower limit of permeate gap MD performance. CGMD and DCMD can also be resilient to high feed salinity by using a thicker membrane. A framework is proposed for choosing the cost-optimal membrane thickness along with optimal membrane size, as a function of the relative cost of membrane area compared to thermal energy. At small system size, increasing area per unit feed flow rate (specific area) leads to better GOR at the expense of lower flux. Beyond a critical specific area, both flux and GOR start to decline. At high feed salinity, this critical area is small enough to be practically relevant. A closed form expression for this critical specific area is derived using the HX analogy equations, as a function of feed salinity, top temperature and the two dimensionless parameters defined previously. Finally, two methods of achieving high overall pure water recovery using membrane distillation, namely batch recirculation and multi-effect MD (MEMD) are analyzed. A batch design outperforms other brine recirculation alternatives such as semi-batch and continuous recirculation. The number of effects is an additional degree of freedom in MEMD system design. The number of effects is analogous to the specific area of single stage MD and plays the major role in determining GOR and flux, whereas the total specific area of MEMD determines its overall recovery ratio. For a given brine concentration application, it is shown that MEMD with feed preheating energetically outperforms batch MD.
Description
Thesis: Ph. D., Massachusetts Institute of Technology, Department of Mechanical Engineering, 2017. Cataloged from PDF version of thesis. Includes bibliographical references (pages 265-276).
Date issued
2017Department
Massachusetts Institute of Technology. Department of Mechanical EngineeringPublisher
Massachusetts Institute of Technology
Keywords
Mechanical Engineering.