Optimal docking to tumbling objects with uncertain properties
Author(s)
Sternberg, David Charles, Ph. D. Massachusetts Institute of Technology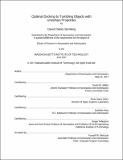
DownloadFull printable version (8.130Mb)
Other Contributors
Massachusetts Institute of Technology. Department of Aeronautics and Astronautics.
Advisor
David W. Miller.
Terms of use
Metadata
Show full item recordAbstract
Active debris removal (ADR), on-orbit servicing and in-space assembly likely require proximity operations and soft docking between two or more satellites. These operations can be complicated by the presence of several challenges, including 1) flexible dynamics, 2) impending collision with other proximity objects, 3) uncooperative satellites, 4) tumbling behavior, 5) uncertainty in the knowledge of this tumble, and 6) the need to maneuver at least one of the satellites through an accelerated approach trajectory. This thesis considers challenges 3 through 6 by addressing the terminal approach and soft docking by a Chaser satellite to a passively tumbling, rigid-body Target. The terminal approach is characterized by the Chaser starting at an initial position several meters away from the Target and aligned with the Target's docking port. It then initializes its inward motion relative to the Target to maintain its position synchronous (i.e., maintaining alignment with the body-fixed frame of the Target's docking port) with the tumbling motion. Using a fuel-efficient trajectory, it traverses along the docking port's body-fixed approach axis by creating the requisite tangential and radial accelerations associated with the Target's tumble. Finally, the Chaser docks with a sufficiently low impact velocity to avoid damage to itself or the Target. This thesis addresses three important aspects of this Synchronous Docking to a Tumbling Target (SDTT) problem, with examples drawn from this ADR mission scenario. First, a methodology is developed to define whether a Chaser can feasibly execute this terminal approach to a Target when that Target's tumbling motion is measured a priori with some known degree of uncertainty. Given the potential urgency in deriving the approach trajectory, a robust and computationally efficient minimum fuel trajectory solver is formulated. This solver is based upon the recognition that fuel optimality requires that the tangential and radial accelerations of the Chaser be equal as it traverses the terminal approach. Since these accelerations are dictated by the Target's uncertain tumbling motion, a Multiple Model optimization technique is used to provide robustness in the trajectory. The result is a methodology that can assess the feasibility of achieving a soft dock of a known Chaser with a Target whose motion is uncertain. Second, this feasibility framework can be used to analyze two Chaser scenarios. The first entails the design of a new Chaser that can capture a broad sub-set of the Target population (Market Capture), while the second entails the determination of the fraction of a Target population, with uncertain dynamics, to which an existing Chaser can feasibly soft dock (Market Analysis). Both scenarios consider levels of uncertainty in the knowledge of the motion of individual Targets in the population that is inherently associated with remote sensing. Third, 0-g simulations are required to obtain predictions of Chaser performance, since this SDTT problem leads to Chasers that conduct multi-axis accelerations uncharacteristic of conventional satellites. A simulation framework that supports both unconstrained 0-g and constrained 1-g performance prediction to flight is developed. The development and validation of key dynamics and environmental factors for both environments are presented. As a result, this research provides a computationally efficient, low-order solver for rapidly deriving a terminal approach trajectory that is both fuel optimal as well as robust to uncertainty in the measurement of the Target's dynamics. Furthermore, a methodology is provided that permits evaluation of the design of a Chaser that can feasibly dock with a desired fraction of the Target population before committing to the cost and effort of developing that Chaser (Market Capture) as well as those Targets to which an existing Chaser can feasibly dock before committing to the time and fuel consumption needed to rendezvous with those Targets (Market Analysis). Finally, a ground test methodology for Chasers that must execute multi-axis accelerated trajectories is presented to minimize the impact of Chaser 0-g modeling errors on the performance of SDTT operations.
Description
Thesis: Sc. D., Massachusetts Institute of Technology, Department of Aeronautics and Astronautics, 2017. This electronic version was submitted by the student author. The certified thesis is available in the Institute Archives and Special Collections. Cataloged from student-submitted PDF version of thesis. Includes bibliographical references (pages 227-242).
Date issued
2017Department
Massachusetts Institute of Technology. Department of Aeronautics and AstronauticsPublisher
Massachusetts Institute of Technology
Keywords
Aeronautics and Astronautics.