Catalytic upgrading of biomass through the hydrodeoxygenation (HDO) of bio-oil derived model compounds
Author(s)
Shetty, Manish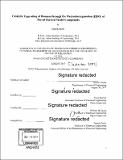
DownloadFull printable version (31.85Mb)
Other Contributors
Massachusetts Institute of Technology. Department of Chemical Engineering.
Advisor
Yuriy Roman and William H. Green.
Terms of use
Metadata
Show full item recordAbstract
Lignocellulosic biomass is an attractive renewable source for fuels and chemicals. Of the many conversion alternatives, catalytic fast pyrolysis has emerged as an attractive technology to convert biomass into fuel additives and value-added chemicals. Current pyrolysis oils or bio-oils are incompatible with refinery streams due to their high acid, water, and water content. The key roadblock in its commercial exploitation is development of catalytic platforms for selective deoxygenation along with minimum hydrogen consumption and carbon loss. Current catalytic solutions including zeolites, and conventional hydrotreating catalysts employ high hydrogen pressures, leading to aromatic ring hydrogenation, and hydrogen consumption. This thesis focusses on developing fundamental catalytic understanding on cheaper and earth-abundant reducible transition metal oxide catalysts for selective hydrodeoxygenation (HDO) of bio-oil derived model compounds using reactivity, computation and characterization studies. The first section focuses on developing structure-reactivity relationships on bulk and supported MoO₃ catalysts for the HDO of lignin-derived model compounds. Characterization reveals that MoO₃ undergoes reduction to catalytically inactive MoO₂ at a temperature of 673 K, and stabilization of partially reduced MoO₃ surface through its partial carburization to oxycarbide phase (MoOxCyHz) at temperatures < 623 K. Thereafter, TiO₂ and ZrO₂ supports prevent the reduction of dispersed oligomeric MoOx species to catalytically inactive species, enhancing their stability. In addition, the overall catalyst reactivity inversely correlates to the maximum hydrogen consumption temperature during hydrogen temperature programmed reduction (H₂-TPR). Furthermore, a near-monolayer oligomeric MoOx dispersion on ZrO₂ support was found to be optimum for HDO reactivity. The second section focuses on developing mechanistic insights into the HDO on bulk and supported MoO₃ with the aid of density functional theory (DFT) computations and kinetic studies. DFT computations were carried out on the elementary steps for HDO of acetone-a model compound on pristine [alpha]-MoO₃ (010) surface to reveal dissociative H₂ adsorption on the (010) surface to be the rate-limiting step. Kinetic studies on MoO₃ supported on ZrO₂ reveal the differences in reaction mechanism and the nature of active sites for HDO on MoO₃/ZrO₂ as compared to bulk MoO₃. The third section focuses on generalizing the low-temperature (< 523 K) selective HDO on other reducible base metal oxides, specifically cobalt oxide and demonstrates oxides to have significantly higher reactivity than base metals for HDO. Finally, lanthanum strontium cobaltite (La₀.₈Sr₀.₂CoO₃), a perovskite oxide, was demonstrated as a novel HDO catalyst at < 523 K. Overall, this thesis provides a toolkit for developing structure-reactivity relationships on reducible metal oxides for their use as HDO catalysts.
Description
Thesis: Ph. D., Massachusetts Institute of Technology, Department of Chemical Engineering, 2017. Cataloged from PDF version of thesis. Includes bibliographical references.
Date issued
2017Department
Massachusetts Institute of Technology. Department of Chemical EngineeringPublisher
Massachusetts Institute of Technology
Keywords
Chemical Engineering.