Multi-scale modeling tools for coupled reaction, phase equilibrium and two-phase mixing phenomena with application to supercritical water heavy oil upgrading process
Author(s)
Raghavan, Ashwin,Ph. D.Massachusetts Institute of Technology.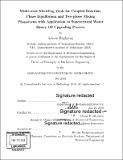
Download1102058215-MIT.pdf (28.21Mb)
Other Contributors
Massachusetts Institute of Technology. Department of Mechanical Engineering.
Advisor
Ahmed F. Ghoniem.
Terms of use
Metadata
Show full item recordAbstract
Supercritical water heavy oil upgrading, which has the potential for high distillate liquid yields with lower coke formation, is a complex process involving coupled reactions, phase equilibrium and two-phase mixing phenomena. This thesis presents the development of a range of models and tools to simulate such processes at different scales with varying levels of fidelity in describing the underlying physical phenomena. Over the past decade, a number of experiments have been performed in batch reactors to study the upgrading of various heavy oils and crude oil vacuum residua through both oil-phase pyrolysis and reaction in the presence of supercritical water (SCW). While these studies indicate that the presence of SCW can significantly affect the outcomes of the upgrading process, there remains a lack of clarity on whether thermolytic processing in the presence of SCW is indeed significantly beneficial as opposed to pure oil phase pyrolysis and if so, at what operating conditions. In addition, modeling tools coupling the reaction kinetics and phase equilibrium, which can provide deeper insight into the process and the underlying phenomena have been lacking. The first part of this thesis describes the development and application of a two-phase stirred reactor (TPSR) model which couples sub-models for the phase-specific reaction kinetics and multi-component hydrocarbon-water phase equilibrium. Using this model, separate lumped kinetics rate parameters for the oil and SCW phases were inferred that best fit batch reactor experimental data. Analysis of the obtained kinetics parameters reveal the following crucial insights on the chemical pathways involved in the SCW upgrading process (i) the primary coke precursor formation pathway is not suppressed in the SCW phase and (ii) only the secondary pathway towards coke precursors from product distillate species is suppressed in the SCW phase, especially at higher operating temperatures. The TPSR model was then applied to evaluate the performance of heavy oil upgrading using SCW in an oil-water co-flow (visbreaking) Next, an extractive upgrading reactor design was hypothesized to improve high-value distillate liquid product yields and reduce undesirable extrinsic coke formation by removing the distillate products safely out of the reactor in a SCW up-flow thereby preventing their further participation in secondary retrograde combination reactions towards more aromatic coke precursors and low-value gas. The TPSR model was used to evaluate the performance of the SCW extractive upgrading process in terms of distillate liquid yields and coke formation rates for heavy oil vacuum residue over a range of operating temperatures and water flow rates. The predictions demonstrate the significant potential of the extractive upgrading process to achieve the aforementioned objectives. The effect of the extraction rate governed by the interphase mixing time-scale on the product yields and oil-inflow rate for steady-state operation was then quantified. The second part of the thesis describes the development of a computational fluid dynamics (CFD) framework and modeling tool for simulating the coupled two-phase flow and multi-component interphase mass transfer at near-critical/supercritical conditions in applications like SCW heavy oil upgrading. The CFD tool accounts for interface tracking in 2-D/3-D, intra-phase species diffusion, phase-equilibrium limited interphase species transfer and non-ideal thermodynamics. In this tool, the interface is tracked with a conservative sharp interface capturing Volume of Fluid (VoF) scheme using (i) a Piece-wise Linear Interface Reconstruction (PLIC) algorithm (ii) an unsplit geometrical advective flux calculation and (iii) a flux-polyhedron correction. The intra-phase species diffusion is handled using a corrected face-normal gradient calculation accounting for the arbitrary shape and size of phase-specific sub-cells. The interphase mass transfer is computed as a source term consistent with the local phase equilibrium and transport flux constraints at the interface. Finally, the phase-volume change is rigorously accounted for in the discrete pressure equation. The tool was implemented on an open-source CFD platform ensuring compatibility with unstructured mesh information and parallel processing constraints. Finally, the developed CFD tool was applied to determine the two-phase mixing rates in an extractive upgrading configuration for water flow rates of interest. The predictions suggest that the earlier assumption of instantaneous phase-equilibration with respect to the time-scale of reactions relevant in the SCW heavy-oil upgrading process is a reasonable approximation for centimeter-scale reactors. Furthermore, the scaling of the total oil-water interfacial area in the reactor and the average Sherwood number with the water inlet velocity and oil-water interfacial tension were established, providing insight into ways to manipulate the two-phase mixing rate to enable control of the extractive upgrading process at higher operating temperatures.
Description
Thesis: Ph. D., Massachusetts Institute of Technology, Department of Mechanical Engineering, 2019 Cataloged from PDF version of thesis. Includes bibliographical references (pages 253-261).
Date issued
2019Department
Massachusetts Institute of Technology. Department of Mechanical EngineeringPublisher
Massachusetts Institute of Technology
Keywords
Mechanical Engineering.