Improving gas fixation in acetogenic bacteria
Author(s)
Emerson, David Frederic.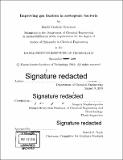
Download1102784561-MIT.pdf (29.10Mb)
Other Contributors
Massachusetts Institute of Technology. Department of Chemical Engineering.
Advisor
Gregory Stephanopoulos.
Terms of use
Metadata
Show full item recordAbstract
Waste gases are an increasing concern for their impact on the environment. This includes carbon dioxide (CO₂) produced from industry and transportation, and methane from distributed oil wells and shale gas formations. Generally, these gases are released to the environment, or CH₄ is burned to CO₂, as CH4 is a more harmful greenhouse gas than CO₂. Researchers are increasingly interested in methods to fix these two gases into valuable fuels and chemicals so as to achieve a sustainable economy. However, any such technology must be economically viable to ensure widespread adoption in the regions that these gases are released. Yet biological gas fixation has many limitations that currently prevent practical implementation. This includes biological limitations such as slow growth rates, and low biomass and product titers. Process limitations are also a concern, as full conversion is desirable and gas mass transfer can be a substantial rate limiting step. Reactors must also be simple and cheap due to the expected cost differential between the reducing equivalents (electricity, H₂ , etc.) and the products (fuels and commodity chemicals). This thesis approaches the limitations of gas fixation from three different perspectives to overcome biological limitations and design low cost bioreactors. Syngas fermentation via the Wood-Ljungdahl pathway is receiving growing attention as a possible platform for the fixation of CO₂ and renewable production of fuels and chemicals. However, the pathway operates near the thermodynamic limit of life, resulting in minimal ATP production and long doubling times. This calls into question the feasibility of producing high-energy compounds at industrially relevant levels. In Chapter 2, we investigated the possibility of co-utilizing nitrate as an inexpensive additional electron acceptor to enhance ATP production during autotrophic growth of Clostridium ljungdahlii. In contrast to other acetogens tested, growth rate and final biomass titer were improved for C. ljungdahlii growing on a mixture of H₂ and CO₂ when supplemented with nitrate. Transcriptomic analysis, ¹³CO₂ labeling, and an electron balance were employed to understand how electron flux is partitioned between CO₂ and nitrate. Finally, we propose a pathway for enhanced ATP production from nitrate, and use this as a basis to calculate theoretical yields for a variety of products. This was experimentally confirmed, whereby nitrate improved heterologous production of 3-hydroxybutyrate, though yields remain much lower than could be theoretically achieved. This work demonstrates a viable strategy for the decoupling of ATP production from carbon dioxide fixation, which will serve to significantly improve the CO₂ fixation rate and the production metrics of other chemicals from CO₂ and H₂ in this host. Future metabolic engineering could greatly increase the yield to those predicted in the theoretical analysis. In Chapter 3, we utilize another electron donor, methanol, for the fixation of CO₂ with the Wood-Ljungdahl pathway. However, the literature is somewhat in disagreement for the mechanism of methanol assimilation by acetogens and the genes involved. Deuterated methanol labeling was used to confirm that methanol was assimilated at the methyl level, indicating that a 3-component methyltransferase system (mtaA BC) was involved. RNAseq analysis revealed that, while mtaB and mtaC were likely properly annotated in Moorella thermoacetica, mtaA was not properly annotated based just on homology. We propose other methyltransferases that were highly upregulated in the presence of methanol as putative mtaA genes that must be confirmed with future in vitro assays. In the final Chapter, 4, we propose a potentially inexpensive reactor for the in situ conversion of methane at natural gas well heads. The mass transfer limits of simple reactor were analyzed, and minimum catalytic rates were calculated such that this methane would be full converted.
Description
Thesis: Ph. D., Massachusetts Institute of Technology, Department of Chemical Engineering, 2018 Cataloged from PDF version of thesis. "September 2018." Includes bibliographical references (pages 193-211).
Date issued
2018Department
Massachusetts Institute of Technology. Department of Chemical EngineeringPublisher
Massachusetts Institute of Technology
Keywords
Chemical Engineering.