Theory of diffusion impedance in nanostructured electrochemical systems
Author(s)
Song, Juhyun.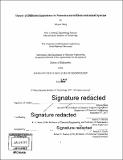
Download1117714958-MIT.pdf (13.23Mb)
Other Contributors
Massachusetts Institute of Technology. Department of Chemical Engineering.
Advisor
Martin Z. Bazant.
Terms of use
Metadata
Show full item recordAbstract
Electrochemical energy systems, including batteries, capacitors, fuel cells, and their hybrids, are under spotlight with ever-increasing interest in their applications to portable electronics, electric vehicles, and stationary energy storage systems. In pursuit of higher energy and power density, as well as longer lifetime, modem electrochemical energy systems commonly employ nanostructured materials: battery electrodes consist of nanoparticles; capacitor electrodes are full of nanopores; and in fuel cells and flow batteries, electrolytes flow through nanoporous electrodes. Their nanostructures determine important parameters affecting the diffusion impedance, such as the diffusion geometries and lengths as well as their distributions. Nonetheless, configuration of the nanostructures is largely overlooked in interpreting the impedance spectra. In this thesis, we investigate the diffusion impedance of nanostructured electrochemical systems by developing theoretical models and validating them with experimental impedance spectra. We begin by studying the diffusion impedance of nanoparticle batteries, and extend the approach to other electrochemical energy systems, including capacitors, fuel cells, and flow batteries. In nanoparticle batteries, the short diffusion lengths of the nanoparticles render the capacitive transition of the bounded diffusion impedance, which is rarely observable with conventional larger particles. The transition can be properly interpreted by considering the configuration of the nanoparticles, and the result presents more precise measurement of the solid-state diffusion than the traditional approach of fitting the Warburg element. A theoretical model incorporating the nanoparticle configuration is developed. Then, by using the model, we investigate the effects of nanoparticle geometry and size distribution on the diffusion impedance. The model is also applied to experimental spectra of a silicon nanowire electrode, and we show that it is essential to take into account the configurational aspects of nanoparticles to correctly interpret diffusion impedance of battery electrodes. Conventionally, diffusion impedance of battery electrodes is interpreted assuming isotropic properties of active particles. While this is reasonable approximation for amorphous or polycrystalline materials with randomly oriented grains, modem battery active materials increasingly consist of highly anisotropic, single-crystalline nanoparticles, for which assuming isotropic properties is not valid any more. Motivated by this trend, we present a general theory of diffusion impedance to include the anisotropic properties in the battery active materials, employing tensorial diffusivities, and orientation-dependent surface properties. Analytical expressions are derived for a single anisotropic particle by finite Fourier transformation. it is then incorporated to the overall electrode impedance by considering the joint distribution of particle lengths in different crystallographic directions. The resulting impedance shows clear signatures of the anisotropic material properties and their statistical variations. In general, nanostructures in electrochemical energy systems have inherent randomness, such as particle size distribution in batteries, pore size distribution in capacitors, tortuosity distribution in membranes and porous electrodes, and inhomogeneous boundary layer thickness in flow batteries. Such configurational randomness commonly introduces the distribution of diffusion time; that is, the diffusion time constant is not set to a single value but rather expands over a range. Therefore, not only nominal properties of the nanostructures, but also their distributions play an important role in diffusion impedance. The finite Warburg and Gerischer impedance models are generalized by incorporating the distribution of diffusion time. Inversion of the model renders accurate image-equivalent information of the nanostructures, suggesting a nondestructive, global diagnostic method, "impedance imaging", by simple impedance measurement. An inversion method based on Tikhonov regularization is presented and demonstrated by applying it to experimental spectra of a capacitor, a battery, and a flow battery. This approach can be generally applied to all electrochemical systems that employing nanostructures.
Description
Thesis: Ph. D., Massachusetts Institute of Technology, Department of Chemical Engineering, 2019 Cataloged from PDF version of thesis. "June 2019." Includes bibliographical references (pages 151-156).
Date issued
2019Department
Massachusetts Institute of Technology. Department of Chemical EngineeringPublisher
Massachusetts Institute of Technology
Keywords
Chemical Engineering.