Towards Impacting Electrochemical Phenomena Using Interfacial Engineering
Author(s)
Khan, Sami,Ph. D.Massachusetts Institute of Technology.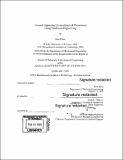
Download1155110779-MIT.pdf (11.00Mb)
Other Contributors
Massachusetts Institute of Technology. Department of Mechanical Engineering.
Advisor
Kripa K. Varanasi.
Terms of use
Metadata
Show full item recordAbstract
Electrochemical phenomena can be broadly defined as any process that involves transfer of electrons, concurrently with a measurable and quantifiable chemical change. Interfacial engineering, on the other hand, can be defined as the altering of surface chemistry and texture across various length scales to impact electro-chemo-mechanical interactions between two or more surfaces. In this thesis, the role of interfacial engineering in impacting two specific electrochemical phenomena has been studied: corrosion and electrochemical reduction of CO 2 In the first part of this thesis, lubricant-impregnated surfaces (LISs) consisting of thin films of lubricant held stably in micro-textures by means of capillary forces have been systematically designed and studied for reducing corrosion. Corrosion is a detrimental process that can impact the performance and lifetime of many infrastructural systems and structures. In this work, we fabricate microposts on silicon using photolithography, varying inter-post spacing systematically from 5 pm to 50 pm, and conformally sputter coat a thin layer of iron to study the corrosion phenomena electrochemically in an aqueous 3.5 wt% sodium chloride solution, and we show that the corrosion rate on LIS is drastically reduced by three orders of magnitude. Using electrochemical impedance spectroscopy, we develop model circuits for various LIS configurations and show that the measured resistances and capacitances agree with a theoretical model, and discuss in detail where deviations may occur. Similarly, we study the role of lubricant layers towards reducing hydrogen embrittlement. Using a Devanathan-Stachurski electrochemical permeation cell we show that under accelerated conditions, the effective diffusion coefficient of hydrogen on steel is reduced by an order of magnitude using LIS. Furthermore, we apply LIS to heal zirconia coatings on steel. Zirconia, that grows natively on zirconium alloys used as cladding for fuel in nuclear reactors, is known to serve as a hydrogen barrier but the presence of grain boundaries or macroscopic surface defects can provide pathways for hydrogen entry. We show that LIS improves the effective diffusion coefficient of a defective zirconia film on steel by an order of magnitude. Finally, we develop aerophilic surfaces to trap CO2 bubbles in close proximity to a CO2 electro-reduction catalyst. Electrochemical reduction of CO2 (CO2RR) to valuable fuels is a promising approach towards reducing the ever-growing atmospheric CO2 levels, and efficient delivery and replenishment of CO2 remains a fundamental challenge that can impact CO 2RR current density and product distribution. When CO2 bubbles are trapped near the catalyst in a plastron layer, the local co 2 concentration available to the catalyst is enhanced and maintained, thereby increasing the magnitude of current density associated with CO2 reduction by close to two times as compared to the conventional CO2 heads pace mode of delivery. We confirm the enhancement in local CO2 concentration using sensitive pH probes as well as colorimetric techniques. Furthermore, we find that hydrogen co-evolution is suppressed from 33% in the heads pace case to 13% with the plastron at -1.1 V vs RHE. We demonstrate that the increase in the CO2RR current density can be scaled up to an array of copper catalysts that possess a higher surface area, while maintaining current density and CO2 concentration close to the catalyst for over an hour. We also demonstrate that CO2RR performance can be enhanced on a porous, nano-structured catalyst using these plastron layers.
Description
Thesis: Ph. D., Massachusetts Institute of Technology, Department of Mechanical Engineering, 2020 Cataloged from PDF version of thesis. Includes bibliographical references (pages 75-86).
Date issued
2020Department
Massachusetts Institute of Technology. Department of Mechanical EngineeringPublisher
Massachusetts Institute of Technology
Keywords
Mechanical Engineering.