Numerical simulation of heavy oil droplets mixing in supercritical water at conditions relevant to supercritical water heavy oil upgrading
Author(s)
Patankar, Aniket Sanjay.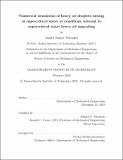
Download1200094458-MIT.pdf (8.551Mb)
Other Contributors
Massachusetts Institute of Technology. Department of Mechanical Engineering.
Advisor
Ahmed F. Ghoniem.
Terms of use
Metadata
Show full item recordAbstract
Supercritical water (SCW) crude oil upgrading is a promising technology for upgrading and desulfurization of heavy crude oil and residues. The outcome of this process is determined by an interplay of hydrocarbon cracking and condensation reactions, multicomponent phase equilibrium, phase separation and coke formation, and macroscale mixing governed by uid dynamics. These individual phenomena span several lengthscales and timescales, and are closely coupled, so that it is extremely difficult to uncover physical phenomena and estimate reaction rates by empirical means alone. Modeling studies of the SCW oil upgrading process are scarce, and pose the challenge of capturing the relevant physical phenomena in a tractable and computable framework. We include a review of SCW-oil upgrading research in the present study, and highlight these modeling challenges. In the present study, we introduce a modeling framework based on a Computational Fluid Dynamics (CFD) scheme developed in-house at the Reacting Gas Dynamics Lab at MIT, and specialized for partially miscible flows with non-ideal thermodynamics. Key features of our CFD scheme include the use of a sharp interface method with geometric advection, and a rigorous treatment of interfacial heat and mass transfer. Preliminary CFD results with a bench-scale reactor geometry are presented to highlight the computational cost of direct numerical simulations (DNS). The novel conditions of SCW oil upgrading result in large Reynolds numbers and very ne droplets at even at low ow velocities. Computational cost can be reduced significantly by developing submodels for estimating mixing rates of oil droplets in SCW. We present high-fidelity, droplet-scale mixing studies under quiescent and convective conditions. Two mixing timescales are quantified for the partially miscible system a droplet saturation timescale and a droplet dissolution timescale. Sherwood number is quantified and droplet deformation and internal circulation within the droplet are examined. Our results suggest that these ow phenomena could lead to significantly altered fractionation and phase separation behavior during convective mixing, as compared to the quiescent case. Equilibrium composition and surface tension vary rapidly with interfacial temperature in the system of interest. We nd that internal circulation within a droplet results in a more homogeneous interfacial temperature distribution. This permits the assumption of a unique equilibrium composition along the interface. However, the strong dependence of surface tension on interfacial temperature could lead to signicant Marangoni forces along the interface.
Description
This electronic version was submitted by the student author. The certified thesis is available in the Institute Archives and Special Collections. Thesis: S.M., Massachusetts Institute of Technology, Department of Mechanical Engineering, 2020 Cataloged from student-submitted PDF of thesis. Includes bibliographical references (pages 251-262).
Date issued
2020Department
Massachusetts Institute of Technology. Department of Mechanical EngineeringPublisher
Massachusetts Institute of Technology
Keywords
Mechanical Engineering.