In vivo translation of near infrared fluorescent semiconducting single walled carbon nanotube sensors : theoretical and experimental applications
Author(s)
Bakh, Naveed Ali.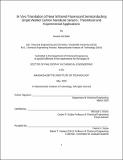
Download1200747232-MIT.pdf (6.080Mb)
Other Contributors
Massachusetts Institute of Technology. Department of Chemical Engineering.
Advisor
Michael S. Strano.
Terms of use
Metadata
Show full item recordAbstract
With the advent of chemical biosensing, a wide variety of biomolecules endogenous to the body are detected in vivo using a number of different methods. Most fluorescent based chemical sensing is primarily done as a form analytical diagnostics, however, recently this technology is being applied to continuous data collection. This work focuses on the translation and usage of fluorescent sensing in vivo, primarily using corona phase molecular recognition (CoPhMoRe) single walled carbon nanotube (SWNT) sensors. These sensors were developed for the lab bench but their translation to in vivo required a number of advances to overcome the method error involved with fluorescent sensor usage in vivo. The goal of this work was to create a framework for the implantation and imaging of intensity modulated sensors to be used in vivo with confidence. This work explored a diverse range of topics in nanosesnsor development and biomedicine. The theoretical and experimental tools developed for this work were then applied to a number of different topics. We initially used pharmacokinetic modeling to predict adipose analyte concentrations for sensor optimization. However, pharmacokinetic model was then adapted to predict glucose responsive insulin (GRI) dynamics. A GRI is a therapeutic that modulates its potency, concentration, or dosing of insulin in relation to a patient's dynamic glucose concentration. Current GRI design lacks a theoretical basis on which to base fundamental design parameters such as glucose reactivity, dissociation constant or potency, and in vivo efficacy. We use well developed pharmacokinetic models of human glucose and insulin metabolism coupled to a kinetic model representation of a freely circulating GRI to determine the desired kinetic parameters and dosing for optimal glycemic control. Our model shows there exists an optimal parameter space that results in successful glycemic control within prescribed constraints over a 24-hour period that persists through a skipped meal. Our results show how tools developed for the sensing space can be applied to adjacent fields. Experimentally, we applied the concept of ratiometric fluorescent sensing to account for the myriad of confounding artifacts that occur while imaging in vivo: from light scattering to mechanical perturbations. The CoPhMoRe technique was applied to find a poly(styrene p-styrenesulfonate) polymer wrapped nanotube for use as a reference and was combined with a DNA SWNT sensor for riboflavin in a hydrogel. The encapsulating hydrogel was shown to preserve the riboflavin sensor response after exposure to 10% mouse serum and after three days of implantation in vivo. Combining the sensor with the invariant reference into a single hydrogel to ratio the modulated signaling, we show that it corrects for in vivo errors, such as breathing and heart-beats, resulting in an order of magnitude increase in confidence signal detection. This works shows the ratiometric hydrogel strategy improves in vivo sensing, enabling SWNT and potentially other fluorescent nanosensor constructs. Even with ratiosensing there is the issue of optimizing the orientation, implantation location, and analyte administration for in vivo imaging of fluorescent sensors, as ratiometric sensing cannot account for all sources of error. We show that subcutaneous implantation and local injection involves significantly more method error compared to intraperitoneal implantation and analyte administration. In combination with hydrogel implants, bottom up imaging, and retro-orbital injections, we show that it is possible to administer analyte systemically while having a stable fluorescent signal. This work has shown the ability to detect local analyte concentrations in vivo with confidence, a potential application of the detection of any generic analyte is introduced as the concept of chemical tomography. Chemical tomography is a technique where a sensor and a tracer analyte can be injected and used to characterize the 3D mass transfer characteristics of a volume. We show proof of concept for using fluorescent nanosensors for vitamins as a way to characterize properties of an environment. In this work we use HUVEC cells adhered to a porous membrane beneath a layer of MoS₂ sensors that change fluorescence in response to riboflavin. By adding riboflavin to this space, we can characterize the 2D diffusivity profile across the porous membrane. Using riboflavin in combination with the sensor we can characterize the decreases in diffusivity as the HUVEC cells contract the space between the pores. Finally, as SWNT sensors are applied to a variety of environments they will inherently experience different laser excitations. Exposing fluorescent SWNT sensors to varying laser fluence [mW/area] can alter their responses to analytes significantly. As the laser fluence increases the nanotube response to certain analytes increases. However, this effect is corona phase dependent as corona phases like sodium cholate and phospholipid-PEG wrapped SWNTs are immune to the range of laser fluences tested. Additionally, we show that increasing the SWNT residence time under laser exposure by encapsulating the sensors in a hydrogel amplifies the effect. Mathematical modeling, Raman G peak shifting studies, fluorescent wavelength shifts all suggest that this effect is not due to laser heating of a single nanotube. We show the importance of testing and accounting for the changes in laser fluence as novel SWNT sensors are developed for new applications.
Description
Thesis: Ph. D., Massachusetts Institute of Technology, Department of Chemical Engineering, May, 2020 Cataloged from the official PDF of thesis. "May 2020." Includes bibliographical references.
Date issued
2020Department
Massachusetts Institute of Technology. Department of Chemical EngineeringPublisher
Massachusetts Institute of Technology
Keywords
Chemical Engineering.