Virus-enabled design of high-performing, three-dimensional nanomaterials for electrochemical energy applications
Author(s)
Records, William Christopher.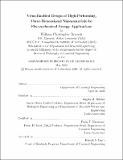
Download1241093847-MIT.pdf (63.67Mb)
Other Contributors
Massachusetts Institute of Technology. Department of Chemical Engineering.
Advisor
Angela M. Belcher and Paula T. Hammond.
Terms of use
Metadata
Show full item recordAbstract
The accelerating pace of anthropogenic climate change has galvanized intensive interest in electrochemical energy storage and conversion. Developing electrode materials for electrochemical devices requires precision and synthetic control over a number of factors, including surface morphology, nanostructure, and distribution of active materials. To this end, my thesis work investigated strategies to implement the M13 bacteriophage as a programmable, lightweight scaffold in the synthesis of three-dimensional, nanoporous foams. Virus-templated nanofoams were incorporated into several relevant energy applications spanning water electrolysis, microbatteries, and electrolytic urea decomposition. The virus-mediated synthesis toolkit yielded clear enhancements in electrochemical performance, as well as design insights into improving nanostructured electrodes in diverse contexts. Virus-templated, platinum-nickel hydroxide nanofoams were first designed and optimized, displaying strong performance as electrocatalysts for the hydrogen evolution reaction in alkaline conditions (ca. -200 mA cm⁻² [subscript geo] and -4.9 A mg⁻¹ [subscript Pt] at -70 mV versus the reversible hydrogen electrode). Mass-normalized activity was definitively linked to the platinum dispersion within the virus-templated matrix, providing a guideline for future electrocatalyst development. Next, virus-templated metal phosphides were engineered with orthogonal control over nanoscale features, phase, and composition. Synthetic versatility was developed across monometallic nickel and copper, as well as bimetallic nickel-cobalt, material systems. When applied as Li-ion microbattery anodes, virus-templated Ni₅P₄ demonstrated a discharge capacity of 677 mAh g⁻¹ (677 mAh cm⁻³) and an 80% capacity retention over more than 100 cycles, outperforming analogous reported Ni₅P₄ materials. The strong performance was attributed to the virus-templated nanostructure, which remains electronically conductive throughout cycling and obviates the need for conductive additives. In the final application, a fundamental exploration into Ni-based catalysts for the electrooxidation of urea was undertaken, highlighting the need for revised benchmarks to facilitate accurate comparisons across the literature and developing an empirical hypothesis for catalyst instability under constant-current electrolysis. Virus-templated, Ni[subscript x]P[subscript y] nanofoams were again applied as electrocatalysts, displaying strong activity relative to the field and enhanced resistance to deactivation. Finally, several directions for scaling methodologies were presented with a future outlook for virus-templating as a material synthesis platform in electrochemical energy storage and conversion.
Description
Thesis: Ph. D., Massachusetts Institute of Technology, Department of Chemical Engineering, May, 2020 Cataloged from student-submitted PDF of thesis. Includes bibliographical references (pages 171-185).
Date issued
2020Department
Massachusetts Institute of Technology. Department of Chemical EngineeringPublisher
Massachusetts Institute of Technology
Keywords
Chemical Engineering.