Engineering gelation in metal ion cross-linked hydrogels
Author(s)
Cazzell, Seth Allen.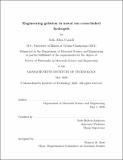
Download1241252165-MIT.pdf (27.66Mb)
Other Contributors
Massachusetts Institute of Technology. Department of Materials Science and Engineering.
Advisor
Niels Holten-Andersen.
Terms of use
Metadata
Show full item recordAbstract
Inspired by their role in the extraordinary mechanical properties of aquatic mussel threads, reversible metal ion cross-links have been utilized to engineer the toughness, stress relaxation, and healing ability of polymer hydrogel networks. Such transient network hydrogels are easily made by reversibly cross-linking a growing variety of polymers modified with ligands capable of binding metal ions in dynamic coordination complexes, and researchers by now have developed a range of orthogonal strategies to tune the viscoelastic properties of these metal ion cross-linked hydrogels. However, several critical challenges have slowed the further development of these materials. Like any two-component cross-linked network, metal ion cross-linked hydrogels are limited by a reliance on strict stoichiometric balance between the metal and ligand to achieve full network connectivity, or percolation, and robust mechanical properties. Additionally, the application space for any hydrogel is ultimately limited by their tendency to either dehydrate or freeze, whereupon the unique material properties that motivated their initial use are lost. Finally, it remains challenging to predict the mechanical properties of metal ion cross-linked hydrogels a priori. This thesis reports new strategies to expand the conditions that allow gelation to occur, create viable materials with a defined application, and predict the mechanical properties of metal ion cross-linked hydrogels. Specifically, we demonstrate that metal ion cross-linked hydrogels avoid traditional stoichiometric limits on gelation through self-regulating hydroxide competition. Additionally, we show that metal ion crosslinked hydrogels can assemble in the presence of large quantities of competitor ligand, further expanding the range of conditions resulting in gelation. Building on these discoveries, we provide a practical demonstration of metal ion cross-linked hydrogels by assembling a broad spectrum vibration damping material, while additionally suppressing dehydration and freezing of the gel. Finally, we develop a computational framework to predict the plateau moduli of metal ion cross-linked hydrogels, a measure of their connectivity and stiffness, as a function of an arbitrary combination of metal ions, ligands, and polymer architecture. The progress made in this thesis should advance the engineering of metal ion cross-linked hydrogels by demonstrating their robust assembly through expanded gelation conditions, their ease of design through our computational model, and their potential application in a broader range of environments due to suppressed dehydration and freezing. More broadly, this thesis pushes forward the development of metal ion cross-linked hydrogels for applications in 3D printing and bioinspired manufacturing and presents a general hypothesis of how to expand gelation conditions in all transient networks outside of metal ion cross-linked hydrogels.
Description
Thesis: Ph. D., Massachusetts Institute of Technology, Department of Materials Science and Engineering, May, 2020 Cataloged from student-submitted PDF of thesis. Includes bibliographical references (pages 195-201).
Date issued
2020Department
Massachusetts Institute of Technology. Department of Materials Science and EngineeringPublisher
Massachusetts Institute of Technology
Keywords
Materials Science and Engineering.