Interfacial and Physical Confinement Effects on the Structure and Properties of Aligned Carbon Nanotube Architectures
Author(s)
Kaiser, Ashley L.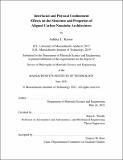
DownloadThesis PDF (238.7Mb)
Advisor
Wardle, Brain L.
Terms of use
Metadata
Show full item recordAbstract
The advantaged mass-specific, intrinsic, and scale-dependent properties of aligned nanofibers, such as carbon nanotubes (CNTs), and their ability to be densified into high volume fraction (commonly, vol%) 3D architectures motivates their use as shape-engineerable materials and composite reinforcement. While controlling nanofiber adhesion to the growth substrate and nanofiber packing density in arrays, e.g. aligned-CNT (A-CNT) arrays in this work, are essential for improving material properties towards bulk-scale manufacturing and application-specific performance, experimental and theoretical approaches to date have not addressed the mechanisms and scaling of CNT-substrate adhesion with processing conditions. Also unexplored is how the nano-, meso-, and micro-scale structures of aerospacegrade composite matrices are affected by high levels of A-CNT confinement (inter-CNT spacings on the order of nm). To understand these effects, this thesis studies the CNTsubstrate strength that governs the manufacturing of shape-engineered CNT arrays, and the interfacial interactions of A-CNTs with the composite matrix that scale performance. In addition to CNT-substrate pull-off tuning via thermal processing, four types of nanocomposites are synthesized to study confinement effects as a function of A-CNT vol%: ACNT-polymer nanocomposites (PNCs) with aerospace-grade epoxy, bismaleimide (BMI), and phenolic matrices, and an A-CNT-carbon nanocomposite (A/C-NC) with a phenolicderived pyrolytic carbon (PyC) matrix.
Thermal post-growth processing of mm-tall aligned CNT arrays on the growth substrate (Fe/Al₂O₃/SiO₂/Si wafers) at temperatures from Tₚ = 700°C (CNT synthesis temperature) up to 950°C in helium for 40 minutes is used to study CNT-substrate pull-off strength. The bulk CNT-array pull-off strength, as measured by CNT array pull-off from the flat substrate via tensile testing, shows that the array fails progressively, similar to microfiber bundles in tension, and in particular evolves non-monotonically with Tₚ with three regimes identified: first increasing from an as-grown strength of ∼0.04 MPa to ∼0.13 MPa up to Tₚ = 735°C (in Regime I), then up to ∼0.35 MPa strength up to Tₚ = 800◦C (in Regime II), and then decreasing back to ∼0.13 MPa strength up to Tₚ = 950◦C (in Regime III). The force-strain relation from tensile testing is modeled analytically based on microfiber bundle mechanics and Weibull statistics, which considers the statistical failure of individual CNTs (fibers) as they either debond from the substrate in Regimes I & II, or break leaving ∼2-micron long CNTs attached to the substrate in Regimes II & III. Morphological and chemical analyses indicate that in all regimes, the Fe catalyst remains on the substrate after CNT array pull-off, and the CNT array structural quality is maintained; higher Tₚ may graphitize the disordered carbon on the substrate and at the CNT roots to increase the substrate adhesion strength for Tₚ up to 800°C, beyond which thermally-induced marring of the substrate is observed in Regime III, where CNT-substrate pull-off strength reduces relative to Regime II.
Key results regarding A-CNT PNCs include process development leading to the first successful fabrication of fully infused, microvoid-free BMI and epoxy PNCs with high volume fractions (1 to 30 vol%) corresponding to square-packing inter-CNT spacings of 70 to 6 nm of mm-tall A-CNT arrays. For both resins, the development of a polymer infiltration model based on Darcy’s law accurately predicts the time for uncured resin to fully infuse into A-CNT arrays during capillary-assisted PNC fabrication, corroborating experimental observations via X-ray micro-computed tomography and microscopy that a diluted 65 wt% resin with ∼10× lower viscosity than neat resin is required for full infusion into dense ACNT arrays (10−30 vol%) for both BMI and epoxy. For each tested vol%, the cured PNCs maintain consistent vertical CNT alignment and glass transition temperature, and the decomposition onset temperature is constant for epoxy PNCs but increases by ∼8°C for BMI PNCs up to 30 vol% A-CNTs. For both polymers, quasi-static nanoindentation yields an ∼2× increase in the axial indentation modulus for 30 vol% A-CNT PNCs compared to the neat resin, with no change in transverse A-CNT modulus, showing enhanced anisotropic mechanical properties with high A-CNT vol%.
Key results regarding A/C-NCs and their phenolic resin PNC precursors include process development leading to the first successful fabrication of fully infused, microvoid-free phenolic 1−30 vol% A-CNT PNCs, and also A/C-NCs after four cycles of polymer infiltration pyrolysis (PIP). A vacuum-assisted thermal drying step is used to remove adsorbed moisture from A-CNTs prior to their capillary-assisted infusion with diluted 50 wt% phenolic resin. The cured PNCs exhibit an 11% increase in decomposition onset temperature and 45% increase in ten-weight-percentage loss temperature from neat resin to 30 vol% PNCs. From PyC to 30 vol% A/C-NCs, 4 PIP cycles decreases porosity by ∼10 vol% and increases bulk density by ∼5% compared to 1 PIP; the 4 PIP A/C-NC bulk density decreases from ∼1.14 g/cm³ to ∼0.80 g/cm³ and porosity increases from ∼47 vol% to ∼74 vol%. Vickers microhardness testing in the axial CNT direction of A/C-NCs shows agreement with prior mechanical modeling and data at low vol% CNTs, where specific hardness increases from ∼3.3 GPa/(g/cm³) for PyC to ∼6.7 GPa/(g/cm³) for 30 vol% A/C-NCs, demonstrating that A/C-NCs are an advantaged superhard lightweight material.
Using the process-structure-property prediction understanding and tools developed in this thesis, more precise tailoring of application-specific performance for aligned CNT architectures is enabled. Future paths of study that enable the design and manufacture of next-generation nanofiber-based materials are recommended.
Date issued
2021-06Department
Massachusetts Institute of Technology. Department of Materials Science and EngineeringPublisher
Massachusetts Institute of Technology