Understanding and Exploiting Anion Redox Process for High Energy Density Positive Electrode Materials for Li-ion Batteries
Author(s)
Yu, Yang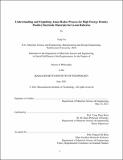
DownloadThesis PDF (30.83Mb)
Advisor
Shao-Horn, Yang
Terms of use
Metadata
Show full item recordAbstract
The development of (hybrid) electric vehicles calls for a better solution for energy storage systems. Li-ion battery system give rise to such opportunity by providing reasonable cycle life and energy density. It was widely assumed that Li-ion battery positive electrode materials, Li transition metal oxides, store charges through the redox activity of transition metal species, accompanied by the intercalation and deintercalation of Li-ions into and out of the host structure. Layered lithium nickel, manganese and cobalt oxides (NMC) are state-of-the-art commercial positive electrodes in the past decades, which relies on the redox of Niᶟ⁺ᐟ⁴⁺ upon charging, limiting further increase of energy density of current Li-ion battery systems. Anionic redox in positive electrode materials in Li-ion batteries provides an additional redox couple beyond the conventional metal redox, which can be harvested to further boost the energy density of current Li-ion batteries. However, the physical origin of observed anion redox remains debated, and more direct experimental evidence is needed. Furthermore, the requirement for the reversible anionic redox activity remains under debate, hindering rational design of new materials leveraging reversible anionic redox.
In this thesis, we primarily focus on understanding the cationic and anionic redox process in the positive electrode materials upon lithium deintercalation using X-ray absorption and emission spectroscopy (XAS and XES), X-ray photoelectron spectroscopy (XPS), coupled with density functional theory (DFT) calculations. We have shown electronic signatures of oxygen-oxygen coupling, direct evidence central to lattice oxygen redox (O²⁻/(O₂)ⁿ⁻), in charged Li₂₋ₓ RuO₃ after Ru oxidation (Ru⁴⁺/Ru⁵⁺) upon first-electron removal with lithium de-intercalation. This lattice oxygen redox of Li₂₋ₓ RuO₃ was accompanied by bulk Ru reduction. This observed redox trend is in stark contrast of the observations in charged Ni-rich NMC upon charging. In Ni-rich NMC positive electrodes, nickel oxidation is primarily responsible for the charge capacity up to removing ~0.7 Li, beyond which was followed by Ni reduction near the surface (up to 100 nm) due to oxygen release, where there is no significant bulk metal reduction observed. The uniqueness of Ru-based system lies in the highly covalent nature of Ru-O bond, stabilizing (O²⁻/(O₂)ⁿ⁻) intermediate, forbidding further oxygen release.
Through systematic transition metal substitution, we have proposed an electronic structure descriptor based on the energetic overlap between transition metal and oxygen to tune the cationic and anionic redox process in Ni-rich NMC as well as Li-rich positive electrode materials to enhance their cycling stability. We have also shown that the electronic structure descriptor can be applied to various electrochemical systems going through redox processes. Our study has laid a solid foundation for future high-throughput screening of novel and affordable metal oxides for battery and electrocatalysis applications.
Date issued
2021-06Department
Massachusetts Institute of Technology. Department of Materials Science and EngineeringPublisher
Massachusetts Institute of Technology