Materials Design for Advanced Nuclear Energy Systems: Refractory High Entropy Alloys and Metallic Multilayer Composites
Author(s)
McAlpine, Samuel W.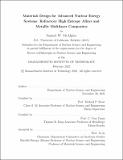
DownloadThesis PDF (146.6Mb)
Advisor
Short, Michael P.
Terms of use
Metadata
Show full item recordAbstract
Advanced nuclear reactors present a multitude of materials challenges due to high op- erating temperatures, corrosive environments, and neutron radiation damage. In this thesis, I focus on two approaches to designing better materials for advanced reactors, high entropy alloys (HEAs) and metallic multilayer composites (MMLCs). HEAs are chemically disordered solid solutions combining 4-5 or more elements, which of- ten have superior mechanical properties and radiation damage tolerance compared to advanced steels and Ni-base alloys. While HEAs have garnered immense attention within the research community, there is still no effective approach for predicting which compositions will tend to form a single phase microstructure. I develop an atomistic thermodynamic model which uses a quantity I coin as the atomistic mixing energy (AME) to understand phase stability in HEAs and predict which elements are more or less favored to mix within a given HEA system. The model also facilitates the correct calculation of the vacancy formation energy distribution in HEAs which gives insight to radiation damage, solid–state diffusion, and other vacancy–driven material behavior. To test the validity of the model, I synthesize and characterize 5 refractory HEA compositions: NbMoTaTiW, NbMoTaTiV, NbMoTaTiZr, NbMoTaHfW, and WTaVTiCr. Implications for single phase HEA design utilizing the model developed in this thesis are explored. The final part of the thesis focuses on MMLCs, in which different material functionalities are separated into different layers. Currently, few studies have aimed to understand radiation damage effects at the interface between different layers. I use interfacial self–ion irradiation along the bimetal interface within 2 MMLC systems to shed light on the radiation damage behavior of the interfacial region. Radiation–enhanced diffusion was observed in one MMLC, and a Cr-rich phase is observed along the interface in both MMLCs. The propensity for radia- tion–enhanced diffusion is related to the compositional gradient across the interface, while the Cr-rich interfacial phase could potentially lead to material embrittlement within MMLCs.
Date issued
2022-02Department
Massachusetts Institute of Technology. Department of Nuclear Science and EngineeringPublisher
Massachusetts Institute of Technology