Experimental Investigation of Critical Heat Flux Enhancement on Engineered Surfaces with Infrared Thermometry
Author(s)
Wang, Chi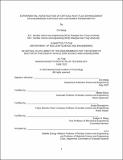
DownloadThesis PDF (6.542Mb)
Advisor
Bucci, Matteo
Terms of use
Metadata
Show full item recordAbstract
The boiling crisis is one of the most intriguing and impactful open problems in thermal science and engineering. The prediction and enhancement of the heat flux at which this boiling crisis occurs (also known as critical heat flux, or CHF) has been the focus of boiling heat transfer research for over a century, as CHF sets an upper limit to the operating heat flux for applications cooled by boiling heat transfer. Surfaces engineered with microscale and nanoscale features have shown great potential to enhance CHF. However, while many hypotheses have been formulated, the mechanisms leading to such enhancement are still debated. This knowledge gap arises from the lack of measurements, e.g., of time-dependent local heat flux and temperature distributions, through which it would be possible to verify hypotheses and modeling assumptions.
This work contributes to removing this technical roadblock and provide new, unique insights into the boiling phenomena and the boiling crisis on surfaces with engineered hydrophilic micropillars.
To achieve these goals, we have developed a special heating surface enabling the use of high-resolution infrared thermometry to obtain the time-dependent temperature and heat flux distribution on the boiling surface. Thank to this technical development, we have been able to collect first-of-a-kind data and shed light on the phenomena involved in the pool and subcooled flow boiling of water on these surfaces, including above-atmospheric pressure conditions (4 bar).
We show that the presence of an intra-pillar liquid layer is the primary reason for CHF enhancement on surfaces with engineered micro-pillars in both pool and flow boiling conditions. However, the energy removed by evaporation of this liquid layer is small compared to the CHF enhancement, e.g., only 30% is removed by evaporation in pool boiling condition. Instead, by delaying the formation of dry spots, the intra-pillar liquid layer empowers other heat transfer mechanisms, e.g., forced convection and transient conduction, to remove more energy from the surface.
This intra-pillar liquid layer consists of two comparable contributions. Initially, liquid is trapped within the pillars as vapor bubbles grow on top of them. Then, liquid is resupplied by capillary wicking effects. The experimental results show that wicking is only effective within a certain distance (<1 mm) from the apparent triple contact line. This wicking distance, is found to depend on the local heat flux which is limited by thermal diffusion in the heater substrate. We can reproduce these observations with mechanistic models describing the evaporation of the intrapillar liquid layer and the dynamic of the flow wicked by the micro-pillars.
Date issued
2022-05Department
Massachusetts Institute of Technology. Department of Nuclear Science and EngineeringPublisher
Massachusetts Institute of Technology