Chemistry, Transport and Function of Ionic Phases in the Solid Electrolyte Interphase on Lithium Metal Anodes
Author(s)
Guo, Rui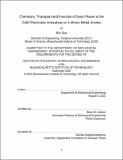
DownloadThesis PDF (6.048Mb)
Advisor
Gallant, Betar M.
Terms of use
Metadata
Show full item recordAbstract
Lithium (Li) metal anodes in liquid electrolytes suffer low Coulombic efficiency (< 99.9%) arising from the chemically inhomogeneous nature of the native solid electrolyte interphase (SEI), which impedes smooth Li plating and leads to excessive electrolyte consumption. Despite much attention paid to engineering Li interfaces of late, there is still limited understanding of the desired chemical composition and structure of an improved Li SEI. One major challenge has been a lack of empirical data on individual SEI phases present at a metallic Li interface, where the chemistry, transport properties and functions have not been well resolved. Consequently, the field still relies on the properties of bulk analogue materials typically invoked to understand SEI behavior.
To address this challenge, this thesis adopted an approach to deconstruct the multiphasic native SEI by building single-component SEI models on metallic Li and probe the transport properties and electrochemistry of individual phases in typical battery electrolytes. In the first part of this thesis, single-component SEI of lithium oxide (Li2O) was synthesized directly onto Li foils by controlled metal-gas reactions, generating model Li2O SEI with nanoscale thicknesses (20–100 nm) commensurate with the native SEI derived from electrolytes. The model Li|Li2O electrodes serve as a platform for further chemical and electrochemical characterizations. In particular, electrochemical impedance spectroscopy (EIS), combined with interface modeling, was used to extract transport properties (i.e., ionic conductivity, diffusivity, charge carrier concentration and activation energy barriers) of Li2O SEI in a carbonate electrolyte. The Li2O SEI was also studied as a function of synthesis conditions, revealing microstructural sensitivities that can be tuned to modulate transport behaviors, and was further compared with similarly deconstructed Li|LiF interfaces to isolate chemistry-specific differences. Our results showed that the ionic conductivity of Li2O model SEI was several orders of magnitude higher than reported values obtained from bulk pellet measurements, revealing dramatically different chemical and microstructural environments between bulk materials and SEI phases.
While chemical evolution of the SEI has been widely recognized in aging processes of Li-ion batteries, pinpointing the chemical origins by tracing them to specific SEI phases has been experimentally challenging. In the second part of this thesis, the single-component model interfaces were further used to study the chemical reactivity between individual SEI phases and battery electrolytes. The degree of interactions between SEI and electrolytes were examined by EIS in the lower-frequency range, and further characterized by X-ray photoelectron spectroscopy (XPS) and X-ray absorption near-edge spectroscopy (XANES). Contrary to some conventional wisdom that ionic phases are stable, our findings shed light on the fact that the ionic SEI phases, particularly in certain electrolytes, can undergo dynamic chemical evolution. These changes can then significantly influence transport through the interfaces in ways that decrease the stability of the SEI. More broadly, this work may have direct implications for ex situ modification approaches for Li: it is critical to examine the reactivity of such interfaces with the electrolyte to ensure that modified interfaces are truly protective and stable.
In spite of the transport properties and chemical reactivity of individual SEI phases (i.e., Li2O and LiF) acquired from single-component SEI studies, there still exists a knowledge gap in understanding the structure-related effects of SEI phases. One particular component of interest is LiF, which has been widely reported as beneficial to an improved SEI, however, the functionality of nanostructured LiF phases in native SEI remains vague. The last part of this thesis examined if and how LiF influences Li deposition under well-defined and experimentally observable conditions. To do so, nanoscale LiF particles with tunable sizes (30–300 nm) were synthesized on Cu electrodes via controlled electrochemical reduction of fluorinated gases. The impact of LiF phases on the overpotential and morphology of Li deposition was studied in battery electrolytes using cyclic voltammetry combined with electron microscopy. Our findings suggested that the total surface area of ex-situ formed LiF particles has a strong positive correlation with the reduction of Li plating overpotential. Hence, this work implied that the morphology of LiF particles in SEI can affect Li nucleation without having significant impacts on the reversibility of Li.
Date issued
2022-09Department
Massachusetts Institute of Technology. Department of Mechanical EngineeringPublisher
Massachusetts Institute of Technology