Simulating, Controlling, and Understanding Lithium-ion Battery Models
Author(s)
Berliner, Marc Dylan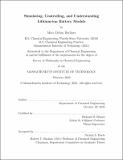
DownloadThesis PDF (7.696Mb)
Advisor
Braatz, Richard D.
Terms of use
Metadata
Show full item recordAbstract
Lithium-ion batteries are widespread in consumer electronics, electric vehicles, and grid storage. Developing better batteries and more intelligent battery management systems is an active area of research – operating batteries safely, maximizing their lifetimes, and inventing new materials are essential for their continued proliferation. To create the next generation of batteries, researchers must tackle challenging, multidisciplinary problems with an enormous design space.
Experimentally testing batteries can be expensive and time-consuming. Extensive analyses usually involve dozens of batteries that may be tested continuously over several weeks or months. Efficient physics-based and data-driven modeling can significantly reduce the cost and time requirements of battery development. Still, physically accurate simulations face numerous technical and theoretical barriers stemming from difficulties in measuring and analyzing battery internals during cycling.
This thesis focuses on simulating, controlling, and understanding rigorous physics-based lithium-ion battery models. The first Part of this thesis presents PETLION, an open-source, high-performance implementation of the Porous Electrode Theory (PET) model. PET typically contains several hundred nonlinear differential-algebraic equations (DAEs) after discretization. This package is designed from a systems engineering perspective to be robust and highly efficient, about 100–1000x faster than other available implementations of PET while maintaining the same physical accuracy. PETLION is the cornerstone for the following Parts of this thesis, permitting deep analyses of PET which would otherwise be prohibitively expensive.
The second Part of this thesis investigates a mixed continuous-discrete (hybrid) approach for fast charging of batteries in real-time. Traditional fast charging problem setups perform optimal control with a reduced-order/empirical model to find the optimal current profile, maximizing the capacity subject to safety and degradation constraints. Instead, a hybrid charging procedure is proposed, simultaneously solving the battery system of equations and the embedded solution to the constraint-based control problem. Here, the fast-charging current profile is found via direct simulation, which dynamically switches between active path constraints. Novel op
Date issued
2023-02Department
Massachusetts Institute of Technology. Department of Chemical EngineeringPublisher
Massachusetts Institute of Technology