Systems Engineering for Viral Vector Manufacturing
Author(s)
Nguyen, Tam Ngoc Thanh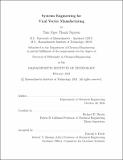
DownloadThesis PDF (18.17Mb)
Advisor
Braatz, Richard D.
Terms of use
Metadata
Show full item recordAbstract
Gene therapies have emerged in recent years as a great promise for treatments against a variety of otherwise difficult to treat diseases. Recombinant adeno-associated virus (rAAV) is the current vehicle of choice for in vivo gene therapy treatments for its reduced toxicity, robust and long-term transgene expression. Rapidly rising interests in both the academic and private sectors have led to increasing demand for gene-therapy products with more than 200 ongoing global clinical trials. The large demand has created a need for manufacturing large quantities of rAAVs. However, current state-of-the-art viral vector manufacturing methods still fall short of meeting current and future demands. Transient transfection via triple transfection of plasmids is the most common manufacturing platform for the clinical stage due to its flexibility in changing the plasmids and transgene.
Triple transfection has low yields and low fraction of full capsids in harvest. The low percentage of full capsids in harvest (1) leads to high doses which could trigger immunogenecity reaction in patient and (2) requires extensive purification which leads to increased development product cost. In order to advance gene therapy development and improve patient accessibility to the therapeutic, manufacturing must be advanced.
At the current state of process development in rAAV vector manufacturing, batch mode is still the method of choice and evaluating the impact of system inputs on the outcome still relies on the empirical approach. Statistical and empirical approaches, such as design of experiment, can be straightforward for optimizing processes but only within a defined parameter space, whereas mechanistic models can provide physical insights and understanding of the process, multivariable interactions and dynamics to guide novel production design, and extrapolated hypotheses that can be tested. While rAAV production is still mostly performed in batch mode, continuous processing can largely reduce footprint of scale-up and provide a much higher degree of flexibility, and remains unexplored for transient transfection to produce rAAV. In this thesis, I presented a step-by-step model-based approach in creating novel methods to produce rAAV vectors, which culminated in a continuous manufacturing platform.
A quantitative analysis of the system via a mechanistic, mathematical model is useful to organize and exploit information obtained from existing data, gain understanding of process dynamics, and predict responses to various process inputs. This thesis constructs a mechanistic, single-cell, triple-transfection model based on AAV replication biology that enables the comprehensive understanding of viral vector production kinetics and facilitates design of processes.
As derived from mechanistic understanding of viral production process, the rapid saturation of the rate of viral capsid synthesis combined with the late onset of viral genome replication could be responsible for the low ratio of full capsids at harvest. This thesis designs a multi-stage transfection strategy in fed-batch that extends the viral capsid production timeline to improve the ratio of full to empty capsids, while keeping the total plasmid dosage constant. The multistage transfection method improved the ratio of full capsids in the harvest while preserving the genome titer.
Continuous processing provides great advantages over batch and fed-batch operations in flexibility and foot-print reduction of scale-up. This thesis develops a perfusion bioreactor platform to produce rAAV at high cell density leveraging understandings from the mechanistic model and multi-stage transfection. The continuous process enables high cell density transfection and reduces genome titer yield per unit weight plasmid.
Date issued
2023-02Department
Massachusetts Institute of Technology. Department of Chemical EngineeringPublisher
Massachusetts Institute of Technology