Engineering topology and correlation in epitaxial thin film kagome metals
Author(s)
Han, Minyong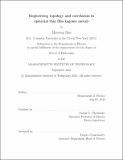
DownloadThesis PDF (12.20Mb)
Advisor
Checkelsky, Joseph G.
Terms of use
Metadata
Show full item recordAbstract
Along with the Landau paradigm of phase transitions, topology has been recognized as an important metric of classifying condensed matter systems, offering successful descriptions for systems with nontrivial energy gaps or protected band degeneracies. As much as topology has risen in significance, the study of correlation has also provided crucial insights for a number of may-body phenomena realized when the inter-particle interactions dominate the kinetic energies of individual constituents. The kagome lattice is a generalized lattice model whose characteristic atomic arrangement produces both topological Dirac bands and correlated flat bands. In search of its material realizations, kagome metals, a class of intermetallics containing the two-dimensional kagome networks of transition metal atoms, have shown promise in faithfully manifesting the original lattice model in their bulk electronic structures.
In this thesis, we present our works on engineering topology and correlation in two representative kagome metals, FeSn and Ni3In, stabilized in epitaxial thin film form. We characterize via transport, thermodynamic, and spectroscopic probes the emergent quantum phenomena arising from the key band structure singularities and further exploit various thin film tuning parameters to manipulate them. With systematic control of chemical potential and spin structure, we elucidate the pivotal roles of the lattice-driven Dirac and flat bands in generating magnetic instabilities and topological edge modes. We also demonstrate how the kagome spectrum reconstructs upon intense inter-kagome hybridization or broken crystallographic symmetry, eventually giving rise to new types of flat bands and their derived anomalies. To track the origins of these, we incorporate films into spintronic or ion-battery devices and monitor their responses under different conditions. These results will construct an important framework in designing topological and correlated electronic states and further driving them towards the regime suitable for functional device applications.
Date issued
2022-09Department
Massachusetts Institute of Technology. Department of PhysicsPublisher
Massachusetts Institute of Technology