Miniature bioanalytical assays utilizing mechanical actuation of microspheres
Author(s)
Hanhauser, Emily B.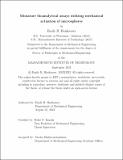
DownloadThesis PDF (58.80Mb)
Advisor
Karnik, Rohit N.
Terms of use
Metadata
Show full item recordAbstract
An estimated 70% of medical decisions rely on diagnostic results, yet half of the global population lacks access to diagnostic testing. Geography contributes to this gap, with decreased access found in decentralized settings, which can lack the physical and labor infrastructure necessary for gold standard bioanalytical assays. In such settings, lateral flow affinity assays remain the technique of choice, due to their fast time-to-result, ease-of-use and deliverable geometry. However, these assays suffer in sensitivity, with limits of detection 10 - 1000x higher than their gold standard counterparts. Capitalizing on transport advantages at small scales, many microfluidic devices enable high sensitivity assays in a compact form, but their translation to the field is often challenging due to their complexity and the required supporting instrumentation. As a result, gaps in diagnostics, suitable for the realities of operating in decentralized settings, remain.
Inspired by challenges demonstrated during the height of the COVID-19 pandemic, this thesis introduces an affinity assay platform that utilizes simple mechanical, nonfluidic actuation of microspheres on a sensing surface to quantify bioanalytes in liquid samples. The assay integrates two processes, capture of analytes during bead sedimentation, and analysis based on bead interactions with a sensing surface using optical microscopy and image processing. The assay can be performed in a standalone device using minimal manual steps and in less than 30 minutes. Combined with single molecule sensitivity demonstrated by previous bead-based assays, this platform has the potential to enable high sensitivity bioanalysis with the ease-of-use profile required for decentralized settings.
In the first part of this thesis, we describe a framework for predicting bead-analyte capture over a range of bioanalytes to aid in design across applications. We apply this method to design and analyze our proposed capture method, and to examine and predict transport advantages that arise from settling microspheres. In the second part, we investigate multiple simple actuation mechanisms that lead to bioanalyte quantification based on bead-surface interactions. While nearly all mechanisms show initial efficacy, thermal diffusion was selected for further development, with demonstrated picomolar limits of detection using a model assay, and bead sliding is also explored for its potential versatility and simplicity of imaging. As nonspecific binding of beads to the surface limits sensitivity, we also theoretically model and experimentally investigate surface coating techniques to minimize this effect and demonstrate preliminary enhanced assay sensitivity using a zwitterionic coating.
Finally, using a simple device prototype, we demonstrate the ability of our assay to quantify cardiac troponin I (cTnI), an established biomarker for cardiac injury. Because elevated levels can indicate heart attack or other critical conditions, measuring cTnI accurately and rapidly is essential. cTnI is quantified via immunoaffinity techniques, with current generation high sensitivity lab assays reaching 0.001 ng/mL limit of detection (LOD) in 30 minutes to one hour and bedside point-of-care (POC) devices having 20x higher LOD but in 15 minutes. The instrumentation requirements of these assays make them expensive and challenging to perform in decentralized settings. As the global leading causes of death continue to transition from infectious to chronic diseases, increasing access to cTnI diagnostics will be crucial to improving health outcomes.
Our results show that our integrated assay can detect cTnI at 0.01 ng/mL in buffer and at 0.1 - 1 ng/mL in 10x diluted serum in a 1% BSA buffer in under 30 minutes. This is a clinically-useful result, with our assay showing LODs 5-25x higher than current bedside POC assays and on par with previous generation high sensitivity lab assays (0.1 – 1 ng/mL), but in a format that requires a single manual transfer step and no specialized or dedicated instrumentation. The performance of the current assay is limited by sensing surface variability, which could be improved by optimization of surface chemistry and blocking reagents. Overall, the platform presented in this thesis could enable quantification of bioanalytes at sensitivities approaching current standard methods but in a user-friendly, high-throughput, distributable and rapid format, an important step forward towards filling the gap in technology for decentralized diagnostics and for other monitoring applications, such as those in water and food safety.
Date issued
2023-09Department
Massachusetts Institute of Technology. Department of Mechanical EngineeringPublisher
Massachusetts Institute of Technology