Atomic-Scale Design at the 2D/3D Interface using Electron Microscopy
Author(s)
Reidy, Kate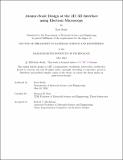
DownloadThesis PDF (169.9Mb)
Advisor
Ross, Frances M.
Terms of use
Metadata
Show full item recordAbstract
Well-controlled nanostructures and their interfaces with surrounding materials are at the core of our most advanced technologies, from superconducting qubits to emerging catalysts. This thesis focuses on elucidating structure, growth, and properties of technologically relevant interfaces between 2D materials and 3D nanoislands, using advanced electron microscopy and spectroscopy techniques. 2D materials represent promising candidates for use in emerging quantum and energy applications, particularly if interfaced in a well-controlled manner with current 3D technological materials, such as conventional metals or semiconductors. At relevant length scales the nanomaterial properties can be altered by the placement of even a single atom or defect in the periodic arrangement of atoms, necessitating the development of new techniques for creating and understanding atomically-precise interfaces.
We begin by proposing general insights for efficient coupling of 2D/3D interfaces at the atomic scale by examining key parameters of growth, including the role of temperature, defects, surface reconstructions, interface chemistry, and thermodynamic equilibrium shapes. We demonstrate how 2D/3D heterostructures grown in ultrahigh vacuum lead to epitaxial, faceted nanoscale islands with ultra-low defect density interfaces. Next, we describe how the interface structure influences local electronic and excitonic properties. We observe reproducible moiré periodicities which vary the electronic charge density, and explain the emergence of an excitonic peak related to the 2D/3D interface via dielectric screening by the 3D contact. We discuss how such understanding of the structure-property-performance relationship allows versatile design of low-dimensional heterostructures for next generation nanoscale devices.
Finally, we extend beyond the 2D/3D metal system to explore further combinations of materials and modes of complex heterostructure design. We fabricate ultrathin multi-element lateral and vertical heterostructures closely linked to future technological integration, and describe how emerging in situ electron microscopy techniques to observe nucleation and growth with high spatial and temporal resolution can unlock fundamental understanding of kinetic and thermodynamic mechanisms, such as growth rates, diffusion phenomena, phase transformation kinetics, strain relaxation mechanisms, and defect formation. The observation of such atomic-scale processes allows us to develop a set of fundamental design rules for building ‘designer materials’ atom-by-atom with tailored properties.
Date issued
2024-05Department
Massachusetts Institute of Technology. Department of Materials Science and EngineeringPublisher
Massachusetts Institute of Technology