Defining Key Engineering Parameters to Advance Electrochemical CO₂ Separation Technologies
Author(s)
Clarke, Lauren E.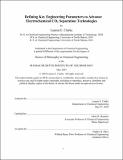
DownloadThesis PDF (42.16Mb)
Advisor
Brushett, Fikile R.
Terms of use
Metadata
Show full item recordAbstract
Carbon dioxide (CO₂) capture, coupled with utilization or storage, is anticipated to help facilitate society-wide decarbonization by reducing emissions of current thermochemical processes and addressing hard-to-decarbonize sectors. However, current carbon capture approaches remain energy intensive, expensive, and dependent on fossil-fuel derived heat, challenging sustainable, large-scale deployment. Electrochemical technologies for CO₂ separation have recently gained attention, as these methods have the potential to achieve higher efficiencies, directly utilize renewable energy, enable modular devices, and operate at (or near) ambient conditions. Various redox chemistries have been identified and experimentally tested for their ability to drive CO₂ separation; however, open questions remain about the expected performance of these systems and how these conceptual processes can be effectively designed at scale. Furthermore, there are several important and interdependent performance descriptors (e.g., energetic efficiency, faradaic efficiency, and separation capacity or flux) whose complex interplay convolutes determination of the optimal design space.
To address this, my research focuses on developing modeling frameworks to better understand the relationship between material properties, operating conditions, and key system performance metrics within electrochemical CO₂ separation systems. First, I discuss a thermodynamic modeling analysis that explores tradeoffs between the upper bounds on energetic and faradaic efficiencies, and identify key molecular/system properties that balance these competing metrics. Then, I describe a cell-level model that was developed to evaluate the impact of several key variables on energetic penalties (on top of the thermodynamics) for liquid-fed electrochemical reactors (used in “4-stage” system configurations). I also demonstrate how this model can be used to explore pathways towards improving the system energetic efficiency. Finally, I describe a 2D model for gas-fed electrochemical cells (used in “2-stage” system configurations) and demonstrate how electrochemically-generated concentration gradients can induce natural convection that can significantly impact observed cell performance. The results from this model highlight how designing cells that harness natural convection can enhance achievable current densities and, ultimately, CO₂ separation fluxes. Overall, the findings from these collective modeling analyses inform ongoing molecular design and device engineering campaigns, provide insight into key system properties, and establish engineering guidelines for electrochemical CO₂ separation devices across scales.
Date issued
2024-05Department
Massachusetts Institute of Technology. Department of Chemical EngineeringPublisher
Massachusetts Institute of Technology