Memory and fluctuations in chemical dynamics
Author(s)
Farahvash, Ardavan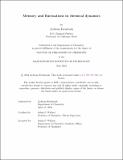
DownloadThesis PDF (12.25Mb)
Advisor
Willard, Adam P.
Terms of use
Metadata
Show full item recordAbstract
This thesis describes the development and application of theories that elucidate both the static and time-dependent responses of various condensed-phase environments to molecular systems. Part I, the cornerstone of this thesis, explores the role of surface vibrations in gas-phase heterogeneous catalysis. Utilizing the Mori-Zwanzig projection operator formalism, I have developed a theory that maps surface vibrations to a generalized Langevin equation (GLE). Two projection schemes are considered. The first scheme projects the motion of the entire solid substrate onto the motion of molecular adsorbates. The second scheme projects onto both the motion of adsorbates and of surface adsorption sites. Through the first approach, I demonstrate that physisorbed species primarily couple with acoustic phonons, while chemisorbed species couple with dispersionless local vibrations. I also use this scheme to examine how phonons affect reactions rates, both in ensembles near and far-from thermal equilibrium. Using the second approach, I study how energy is dissipated in simulations of molecule-surface scattering. I demonstrate that phonon confinement effects from nanoscale simulations can significantly impact calculated surface sticking coefficients. Part II considers the role of solvent in adsorption and desorption at liquid-solid interfaces. Specifically, I employ enhanced sampling methods to study a model system of carbon monoxide at a water/platinum interface. Using these methods, I show that the local coordination number around a CO molecule plays a crucial role in the transition states of the adsorption/desorption process, and that CO tends to increase its coordination number before desorbing. Part III develops a machine learning and electronic structure framework for the computationally efficient parametrization of Frenkel Hamiltonians from snapshots of molecular dynamics simulations of organic semiconductors. Direct electronic structure calculations on these snapshots encode the nuclear fluctuations of the chromophores in the material and how they couple to excitons, but at enormous cost. I discuss how the strategic application of machine learning methods can drastically reduce the number of electronic structure calculations needed to produce a complete exciton trajectory. Critically, I demonstrate that by decomposing the two-molecule excitonic coupling into interactions between one-molecule transition monopoles, a more accurate and less data-intensive machine learning scheme can be devised.
Date issued
2024-05Department
Massachusetts Institute of Technology. Department of ChemistryPublisher
Massachusetts Institute of Technology