Discovering non-equilibrium mechanisms that regulatestructure and function of biomolecular condensates usingphase-field modeling
Author(s)
Natarajan, Pradeep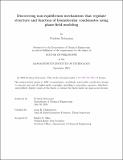
DownloadThesis PDF (19.84Mb)
Advisor
Chakraborty, Arup K.
Terms of use
Metadata
Show full item recordAbstract
Biomolecular condensates are phase-separated assemblies in living cells that form through cooperative interactions between their constituents such as proteins and RNAs. They are emerging as important organizers of biochemistry in cells and are dysregulated in disease. Understanding the physical principles that shape the form and function of these condensates is a fundamental scientific challenge, which if addressed can provide novel therapeutic avenues to improve human health. The equilibrium principles behind condensate formation are well understood. Several studies have investigated the impact of multivalency, protein sequence, protein-RNA interactions, and the role of DNA in modulating biomolecular interactions that drive phase separation. However, the living cell is inherently out of equilibrium with non-equilibrium reactions that constantly burn ATP and turn over biomolecules. My thesis investigates how the interplay between biomolecular interactions and non-equilibrium reactions that turn over biomolecules affect the structure and function of biomolecular condensates. Phase-field modeling is used for these investigations, as this approach has been historically successful in answering similar questions in other fields such as material science. Prior work shows that proteins present in biomolecular condensates associated with RNA transcription undergo complex coacervation with the RNA product. The first project in this thesis investigates the interplay between complex coacervation and spatially heterogeneous RNA synthesis on condensate morphology and dynamics using a phase-field model. This simple model exhibits a rich variety of dynamical behaviors and steady states. It also provides a unifying framework to explain diverse experimental observations related to condensate morphology and dynamics such as vacuole formation, aspherical shapes, directed motion, and splitting-fusion behaviors. The second project investigates how transcription of messenger RNA (mRNA) by transcriptional condensates is modulated by other RNAs in the vicinity, such as long non-coding RNAs (lncRNAs). Our model reveals that lncRNA transcription in the vicinity can regulate mRNA transcription by altering the protein concentration and lifetime of transcriptional condensates, 3 promoting mRNA transcription from genes expressed at a low level and inhibiting transcription from highly expressed genes. This model provides a unifying framework to reconcile conflicting observations in the literature about transcriptional regulation by lncRNAs. The final project focuses on the fibrillar center of the nucleolus, an important condensate that is involved in ribosome biogenesis. Using a phase-field model that explicitly accounts for rRNA-protein interactions in the nucleolus and the non-equilibrium reaction of rRNA transcription, we show that the coarsening of fibrillar centers is arrested leading to a preferred size. Altering this size affects rRNA export and processing from the fibrillar centers. These predictions are validated by experiments. Using a combination of experiments and theory, we uncover the non-equilibrium mechanism that controls size control of fibrillar centers and the functional consequences of this size control on rRNA processing.
Date issued
2024-09Department
Massachusetts Institute of Technology. Department of Chemical EngineeringPublisher
Massachusetts Institute of Technology