On the dynamics and interparticle forces of electrostatically stabilized colloidal suspensions
Author(s)
Krucker-Velasquez, Emily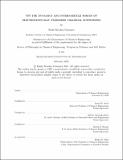
DownloadThesis PDF (33.24Mb)
Advisor
Swan, James W.
Alexander-Katz, Alfredo
Bazant, Martin Z.
Terms of use
Metadata
Show full item recordAbstract
In a broad spectrum of industrial and biomedical applications, the equilibrium and dynamic properties of colloidal suspensions play a pivotal role, with systems ranging from simple gold nanoparticles in electrolyte solutions to complex assemblies like micelles, vesicles, nanocapsules, and dendritic polymers. Typically, these systems are approached through the Derjaguin–Landau–Verwey–Overbeek (DLVO) theory and Poisson-Boltzmann models, frameworks that approximate charged particles as point charges to predict interparticle interactions. While these frameworks have been instrumental for low-concentration, idealized systems, it falls short in capturing critical behaviors in more concentrated regimes. In such environments, overlooked phenomena—such as excluded volume effects and ion-ion correlations—become essential in shaping the colloidal system’s equilibrium and dynamics. By leveraging advanced computational techniques, we systematically interrogate these mesoscale interactions, offering insights that extend beyond the traditional paradigms of mean-field theory and enhance our understanding of colloidal behavior in complex environments. The first part of this work presents the development of efficient algorithms that significantly advance the computational speed of induced polarization calculations within Brownian Dynamics simulations of polarizable colloidal particles. By establishing a new benchmark in simulation methodologies, these algorithms lay the groundwork for exploring complex soft matter systems, enabling deeper insights into the dynamic and equilibrium properties of colloidal suspensions beyond the limitations of conventional theories. Together, these advancements provide a robust computational framework for examining mesoscale interactions in concentrated colloidal systems, where ion correlations, finite ion volumes, and thermal fluctuations critically influence behavior. The next part of this work focuses on the study of equilibrium properties of charged soft matter systems in crowded environments through the implementation of robust computational techniques. We meticulously examine charge-density correlations and clustering behaviors that arise due to the complex electrostatic interactions between colloidal particles. At high ion concentrations, the system undergoes distinct structural transitions that are modulated by the ionic strength and specific particle characteristics. These transitions are characterized by emergent patterns in the spatial distribution of charges, forming structured clusters that reflect the balance between electrostatic and entropic forces. We further our studies by computing the potential of mean force (PMF) between metallic nanoparticles, a measure of the effective interaction potential that inherently captures how particles interact across various separation distances in an electrolyte. The PMF analysis reveals oscillatory behavior in particle interactions at different concentrations. Our study delivers robust free energy profiles, enabling a more nuanced understanding of the electrostatic forces at play in dense colloidal suspensions. These insights shed light on the mechanisms of charge screening and packing within high-density systems. The final part of this thesis focuses on the study of the non-linear transport properties of concentrated macroions to external electric fields, revealing intricate dependencies on both ionic structure and external electric fields. Our studies reveal how conductivity is modulated by charge density correlations and field strength. A notable disruption of local ionic atmospheres was observed with increasing field strengths, which in turn accelerates ion mobility and significantly alters the transport properties. We further advance the investigation into the dynamic response of concentrated macroions and electrolytes by examining their behavior under time-varying electric fields. Through simulations involving frequency sweeps and chirp signals, we discerned that the dynamic response of these concentrated charged soft matter systems is best understood through the lens of two distinct transport regimes—characterized by short- and long-time responses. This bifurcation enables the introduction of a relaxation time scale that captures the intricate coupling between ionic correlations and the macroscopic system response, highlighting the pivotal role of excluded volume effects in densely populated environments. The study provides a detailed framework for manipulating ion transport in concentrated electrolytes and macroions, paving the way for innovations in fields reliant on precise control of electrostatic conditions and ionic mobility.
Date issued
2025-02Department
Massachusetts Institute of Technology. Department of Chemical EngineeringPublisher
Massachusetts Institute of Technology