Mass transfer and structural analysis of microfluidic sensors
Author(s)
Gervais, Thomas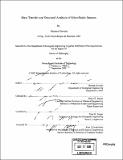
DownloadFull printable version (20.17Mb)
Other Contributors
Massachusetts Institute of Technology. Biological Engineering Division.
Advisor
Klavs F. Jensen and Peter K. Sorger.
Terms of use
Metadata
Show full item recordAbstract
Surface-based sensors take advantage of the natural high surface-to-volume ratios in microfluidic devices, low reagent consumption and high potential for integration in more complex micro total analysis systems (microTAS or pTAS). This thesis studies the fundamental limits of on-chip integrated microfluidic sensors. More specifically, it focuses on detection methods involving surface interaction in channels with thicknesses on the order of a few microns or less. Through mass transfer analysis, we demonstrate that, for thin enough channels, sample detection is limited by the convective transport of analytes, and neither by diffusion nor reaction. The results provided extend the validity of transport models to include transport in the absence of mass transfer boundary layer. All existing analytic solutions to the Graetz problem are described and compiled. The analysis, complemented by finite element simulations, successfully predicts experimental observations made for on-chip immunoassays in micron-thick fluidic channels. Subsequently, our study of on chip detection systems is carried on with emphasis on resonating cantilever sensors. In order to interpret the output signal from these devices, we develop a dynamic cantilever model to link spatially and temporally dependent mass adsorption with resonance frequency change. (cont.) The mass adsorption is then directly related to the sensors' operating conditions via the mass transfer models previously developed. We then develop a 2D finite-element model capable of predicting the devices response and of extracting bimolecular rate constants. Finally, since hydraulic resistance severely increases as channels get shallower, we provide a structural analysis of polymer-based microsystems. Through scaling and numerical simulations we demonstrate the effect of channel deformation on the flow conditions inside the device and vice versa. Finally, channel deformation is experimentally quantified using optical methods and compared with the models developed. Throughout this thesis, the approach to physical modeling has been to use mathematical and numerical analysis as predictive tools in the design of integrated lab-on-a-chip systems. Whenever possible, scaling and analytic solutions are developed, since they provide a direct relationship between experimental observations, geometry and the multiple dependent variables in the system, and can be readily used as design criteria by the experimentalist.
Description
Thesis (Ph. D.)--Massachusetts Institute of Technology, Biological Engineering Division, February 2006. Includes bibliographical references (leaves 181-191).
Date issued
2006Department
Massachusetts Institute of Technology. Department of Biological EngineeringPublisher
Massachusetts Institute of Technology
Keywords
Biological Engineering Division.