Co-oxidation in supercritical water : methylphosphonic acid-ethanol and ammonia-ethanol model systems
Author(s)
Ploeger, Jason M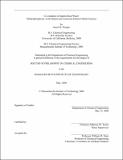
DownloadFull printable version (2.249Mb)
Alternative title
Co-oxidation in SCW : methylphosphonic acid-ethanol and ammonia-ethanol model systems
Other Contributors
Massachusetts Institute of Technology. Dept. of Chemical Engineering.
Advisor
Jefferson W. Tester.
Terms of use
Metadata
Show full item recordAbstract
Supercritical water (SCW) is an effective solvent for the destruction of organic compounds by oxidation. Because both organics and oxygen have high solubility in water above its critical point (To = 374 °C (647 K), Pc = 221 bar), they can be reacted together in a single phase which avoids mass transfer limitations. At typical operating conditions (T= 450 to 650 °C, P = 240 to 300 bar) for supercritical water oxidation (SCWO), H-C-N compounds are rapidly and completely oxidized to water, carbon dioxide, nitrogen, and nitrous oxide. The destruction of organic compounds in SCWO takes place primarily through free radical pathways rather than the ionic pathways that dominate in liquid water. This is because SCW acts as a nonpolar solvent with a dielectric constant ranging from 1.2 at T= 650 °C and P = 250 bar to 2.5 at T= 450 °C and P = 250 bar as compared to ambient water which has a dielectric constant equal to 80. The ion product of water, K, similarly drops to between 10-8 and 102° over this temperature range as compared to the ambient value of 10-14. Typically, SCWO has been studied by the analysis of either the oxidation of single model compounds to determine detailed kinetic mechanisms or by the oxidation of complex simulated waste streams to measure DRE levels. (cont.) While kinetic rates and mechanisms are accurately determined by the analysis of pure compounds, this approach fails to characterize the co-oxidation effect: a phenomenon observed in mixed waste streams where refractory compounds oxidize more rapidly in the presence of labile compounds. The purpose of this research is to provide a quantitative mechanistic understanding of co-oxidation rate enhancement in supercritical water. This understanding is vital for the application of predictive elementary reaction rate models developed for individual model compounds to the analysis of mixed waste streams. By combining the two well-characterized, validated SCWO models for ethanol and MPA, mechanistic insight into the interaction of the two compounds in SCW is possible. This insight into the mechanism of co-oxidation could then be used to assist in the development and validation of an elementary reaction rate mechanism for ammonia. (cont.) The co-oxidative effect of ethanol on methylphosphonic acid (MPA, or PO(OH)2CH3) was characterized for a range of MPA concentrations (0.1 to 1.0 mM) and ethanol concentrations (0 to 2.4 mM) for temperatures of 473 °C and 528 °C, a pressure of 245 bar, and stoichiometric oxygen for the complete combustion of both organic compounds. Low concentrations of ethanol (0.1 and 0.3 mM) were found to have no statistically significant effect on MPA conversion for an initial MPA concentration of 1.0 mM, but higher concentrations of ethanol caused an increase in the conversion of MPA at T= 473 °C, P = 245 bar, and r = 9 s from 14±2% without ethanol present to 29-2% with 1.0 mM ethanol and 39±2% with 2.4 mM ethanol. The increase in MPA conversion was more pronounced at shorter residence times. Decreasing the initial concentration of MPA at a constant initial ethanol concentration of 1.0 mM, T= 473 C, P = 245 bar, and = 9 s resulted in an increase in MPA conversion from 29±2% at 1.0 mM MPA to 41±2% at 0.1 mM MPA. At T = 528 C and P = 245 bar, the initial concentration of MPA had a much greater effect on MPA conversion than the initial concentration of ethanol. (cont.) A supercritical water co-oxidation elementary reaction rate mechanism was constructed from submechanisms for MPA and ethanol with updated kinetic rate parameters for H202 and HOCO' chemistry. The co-oxidation mechanism accurately reproduces the experimentally observed conversion trend of the refractory MPA component as a function of initial concentration of the labile ethanol component. The increase in MPA conversion with increasing ethanol concentration is predicted to be caused by the increased concentration of hydroperoxy radicals (HO2') produced by ethanol oxidation. An analysis of the major organophosphorus reaction fluxes indicated that the co-oxidative effect would increase the conversion of MPA but not change the rate of formation of methane. An experiment using a model formaldehyde/methanol mixture as a co-oxidant was conducted to confirm this prediction. (cont.) The co-oxidative effect of ethanol on ammonia oxidation in supercritical water was studied for a range of temperatures (655-705 C), initial ammonia (1 to 3 mM), ethanol (0 to 1.0 mM), and oxygen concentrations (0.7 to 5.0 mM), corresponding to fuel equivalence ratios ranging from 0.9 to 2.2. With a stoichiometric amount of oxygen available for complete oxidation, the addition of ethanol on an equivalent molar basis was found to increase ammonia conversion from 20% to 65% at initial concentrations of 1 mM for each reactant, T= 700C, P 246 bar, and X = 2.5 s. Nitrous oxide was produced in much larger quantities for ammonia-ethanol co-oxidation than for ammonia oxidation. Based on fractional yields of nitrogen product, this amounted to 40 to 75% for co-oxidation with ethanol versus 4 to 13% without ethanol present. A co-oxidation model was constructed from submechanisms for ammonia combustion at atmospheric pressure conditions and ethanol oxidation in supercritical water. The initial mechanism poorly reproduced experimental ammonia conversion data and was not able to consistently match nitrous oxide production as a function of temperature over a range from 655 to 700 C. In order to improve model predictions, the low-pressure NH2+NOx submechanism was replaced with a submechanism that included the H2NNOx adduct species that are expected to be stabilized in the high-pressure supercritical water environment. Thermochemical and kinetic parameters for the adduct species were estimated with quantum chemical calculations using Gaussian 98 with the CBS-Q method. The explicit treatment of the H2NNOx adducts resulted in nitrous oxide yield predictions that correctly reproduced experimental trends. (cont.) In order to improve model predictions, the low-pressure NH2+NOx submechanism was replaced with a submechanism that included the H2NNOx adduct species that are expected to be stabilized in the high-pressure supercritical water environment. Thermochemical and kinetic parameters for the adduct species were estimated with quantum chemical calculations using Gaussian 98 with the CBS-Q method. The explicit treatment of the H2NNOx adducts resulted in nitrous oxide yield predictions that correctly reproduced experimental trends.
Description
Thesis (Ph. D.)--Massachusetts Institute of Technology, Dept. of Chemical Engineering, 2006. This electronic version was submitted by the student author. The certified thesis is available in the Institute Archives and Special Collections. Includes bibliographical references.
Date issued
2006Department
Massachusetts Institute of Technology. Department of Chemical EngineeringPublisher
Massachusetts Institute of Technology
Keywords
Chemical Engineering.