Electronic structure and quantum conductance of nanostructures
Author(s)
Lee, Young-Su, Ph. D. Massachusetts Institute of Technology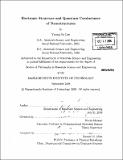
DownloadFull printable version (25.28Mb)
Other Contributors
Massachusetts Institute of Technology. Dept. of Materials Science and Engineering.
Advisor
Nicola Marzari.
Terms of use
Metadata
Show full item recordAbstract
This thesis is dedicated to development and application of a novel large-scale first-principles approach to study the electronic structure and quantum conductance of realistic nanoscale materials. Electron transport at the nanometer scale involves phenomena which are beyond the realm of classical transport theory: the wave character of the electrons becomes central, and the Schrddinger equation needs to be solved explicitly. First-principles calculations can nowadays deal with systems containing hundreds of electrons, but simulations for nanostructures that contain thousands of atoms or more need to rely on parametrized Hamiltonians. The core of our approach lies in the derivation of exact and chemically-specific Hamiltonians from first-principles calculations, in a basis of maximally-localized Wannier functions, that become explicit tight-binding orbitals. Once this optimal basis is determined, the Hamiltonian matrix becomes short-ranged, diagonally-dominant, and transferable - i.e. a large nanostructure can be constructed by assembling together the Hamiltonians of its constitutive building block. This approach is first demonstrated for pristine semiconducting and metallic nanotubes, demonstrating perfect agreement with full first-principles calculations in a complete planewave basis. (cont.) Then, it is applied to study the electronic structure and quantum conductance of functionalized carbon nanotubes. The first class of functionalizing addends, represented by single-bond covalent ligands (e.g. hydrogens or aryls), turns out to affect very strongly the back-scattering and the conductance, since sp3 rehybridization at the sidewall carbon where a group is attached dramatically perturbs the conjugated [pi]-bonding network. Inspection on the shape and the on-site energy of MLWFs before and after functionalizations leads to the conclusion that the effect of sp3 rehybridization is essentially identical to removing a "half-filled" p-orbital from the [pi]-manifold. In this perspective, the chemical difference between functional groups (e.g. different electronegativity of the residues) is relatively minor, even if, of course, will lead to different doping of the tube. We also find that these single-bond ligands tend to cluster, and are more stable when two groups are located nearby (incidentally, the degree of perturbation at the Fermi level becomes weaker when such paired configuration is assumed). The second class of functionalizing addends, represented by cycloaddition functionalizations (e.g. carbenes and nitrenes), demonstrates a radically different behavior. (cont.) These addends are bonded to two neighboring sidewall carbon atoms, creating a three-membered ring structure. On narrow-diameter tubes, cleaving of the sidewall bond takes place to release the high strain energy of a three-membered ring. In the process, the two sidewall carbons recover their original sp2 hybridization. This step is crucial, since the quantum conductance of a metallic nanotube then recovers almost perfectly the ideal limit of a pristine tube: the bond cleavage restores a transparent conduction manifold. Bond cleavage is controlled by the chemistry of the functional groups and the curvature of the nanotubes. High-curvature favors bond opening, whereas in graphene the bond is always closed; in between the two limits, chemistry determines the critical curvature at which the open-to-closed transition takes place. The preference for bond opening or closing has been screened extensively for different classes of functional groups, using initially some molecular homologues of the nanotubes. It is found that a subclass of addends, exemplified by dicyanocarbene, can assume both the open and closed form in the same tube around a narrow range of diameters. (cont.) While these two forms are very similar in energy, and separated by a small barrier (hence they can be considered "fluxional" tautomers), the quantum conductance in the closed case is found to be significantly lower than that in the open case. Interconversion between the two minima could then be directed by optical or electrochemical means, in turn controlling the conductance of the functionalized tubes. We envision thus that this novel class of functionalization will offer a practical way toward non-destructive chemistry that can either preserve the metallic conductance of the tubes, or modulate it in real-time, with foreseeable applications in memories, sensors, imaging, and optoelectronic devices.
Description
Thesis (Ph. D.)--Massachusetts Institute of Technology, Dept. of Materials Science and Engineering, 2006. Includes bibliographical references (p. 145-158).
Date issued
2006Department
Massachusetts Institute of Technology. Department of Materials Science and EngineeringPublisher
Massachusetts Institute of Technology
Keywords
Materials Science and Engineering.