Computer simulation and topological modeling of radiation effects in zircon
Author(s)
Zhang, Yi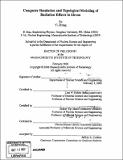
DownloadFull printable version (182.8Mb)
Other Contributors
Massachusetts Institute of Technology. Dept. of Nuclear Science and Engineering.
Advisor
Linn W. Hobbs.
Terms of use
Metadata
Show full item recordAbstract
The purpose of this study is to understand on atomic level the structural response of zircon (ZrSiO4) to irradiation using molecular dynamics (MD) computer simulations, and to develop topological models that can describe these structural changes. Topological signatures, encoded using the concepts of primitive-rings and local clusters, were developed and used to differentiate crystalline and non-crystalline atoms in various zircon structures. Since primitive-rings and local clusters are general concepts applicable to all materials, and the algorithms to systematically identify them are well-established, topological signatures based on them are easy to implement and the method of topological signatures is applicable to all structures. The method of topological signatures is better than the Wigner-Seitz cell method, which depends on the original crystalline reference grid that is unusable in heavily damaged structures or regions; it is also better than those methods based only on local structures limited to first coordination shell, since one can decide whether or not to include ring contents of large rings into the topological signatures, effectively controlling the range of the topological signatures. The early-stage evolution of non-crystalline disorder and the subsequent recrystallization in zircon collision cascade simulations were successfully modeled by using the topological signatures to identify non-crystalline atoms. Simply using the number of displaced atoms was unable to correctly show the initial peak of structural damage followed by the subsequent annealing stage. Using the topological signatures, amorphization within a single collision cascade was observed in zircon. (cont.) In the radiation-induced amorphous zircon simulated in this study, the method of topological signatures was able to differentiate the amorphous region in the center of the simulation box and the crystalline region surrounding it. A few isolated remnant crystalline islands were identified in the amorphous region. About 5% of atoms in melted and melt-quenched structures were identified as crystalline atoms. Different amorphous zircon structures were found to be topologically different. Upon amorphization of zircon, the average ring size and the number of atoms in local cluster were found to increase. Larger average ring sizes were found in more pervasively amorphized structures. The radiation-induced amorphous structure was the least pervasively amorphized one, followed by the melt-quenched. The liquid-state amorphous structure was most pervasively amorphized and had the largest average ring size. Phase-separation of zircon into SiO2- and ZrO2-rich local regions was observed when zircon was amorphized in simulations, either thermally or by radiation. It was found in simulations using constant pressure ensembles that the zircon structure underwent abnormally huge volume swelling when it amorphized, which was attributed to the ion charges used in the potential model. Although the ion charges used in the originally chosen potential model were overall balanced, they were not balanced with regard to the phase decomposition products, and thus resulted in strong Coulombic repulsive force within locally SiO2- and ZrO2-rich regions when phase separation occurred. After the ion charges were re-balanced (and other potential parameters refitted), the volume expansion was found to be under control. The charge imbalance of SiO2 units was also found to produce unrealistically large fraction of 3-coordinated Si and shorter Si-O bond length. (cont.) The issue of charge-balance with regard to phase decomposition products applies to all complex ceramics that decompose into separate phases upon amorphization. Threshold displacement energies in zircon were systematically determined. Many special directions, such as those directed toward neighboring atoms or open spaces surrounding the PKA, were considered. Cascade detail was extensively examined, including PKA trajectory, cascade extent, time scale, thermal spike, recoil density, distribution of PKA energy among sub-lattices and number of displaced atoms. The crystallographic features of the zircon structure were found to have profound implications for collision cascades. It was found that energetic PKAs were always deflected into the open channel along the z direction. Their displacements along the longitudinal x direction were never greater than about 4 nm in our simulations. The estimation of the cascade extent assuming homogeneous media thus greatly over-predicts the PKA displacement along the longitudinal direction. The effects of PKA mass on collision cascade were studied by comparing the cascades caused by Zr and U PKAs. The U atoms were simply "super-mass" Zr atoms in this study: U-Zr, U-Si and U-O interactions were the same as Zr-Zr, Zr-Si and Zr-O interactions, respectively. It was found that heavier PKAs produced longer cascades, more structural damage, and higher temperature in thermal spike. U also traveled further along the longitudinal x direction because it was less prone to change of velocity direction. The depleted regions in the core of the cascades surrounded by a densified shell, which were found in simulations by Trachenko et al., were not found in our study. After extensive tests of recently published zircon potentials, it was found that three out of the five tested potentials yielded poor elastic constants and appear to be unfit for serious simulations. Published simulation results using these potentials should accordingly be viewed cautiously.
Description
Thesis (Ph. D.)--Massachusetts Institute of Technology, Dept. of Nuclear Science and Engineering, 2006. Includes bibliographical references.
Date issued
2006Department
Massachusetts Institute of Technology. Department of Nuclear Science and EngineeringPublisher
Massachusetts Institute of Technology
Keywords
Nuclear Science and Engineering.