High open-circuit voltage in heterojunction photovoltaics containing a printed colloidal quantum-dot photosensitive layer
Author(s)
Arango, Alexi Cosmos, 1975-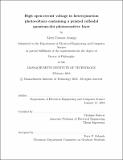
DownloadFull printable version (15.99Mb)
Other Contributors
Massachusetts Institute of Technology. Dept. of Electrical Engineering and Computer Science.
Advisor
Vladimir Bulović.
Terms of use
Metadata
Show full item recordAbstract
Within four to seven years, electricity generated from solar cells will cost less than grid electricity, making it the cleanest, cheapest, and most abundant energy source on the planet. The rise of solar energy, however, could come to an untimely end if current solar cell technologies fail to meet the staggering manufacturing volumes needed to sustain current growth rates. Nanostructured donor/acceptor photovoltaics utilizing small molecule organics or conjugated polymers offer processing advantages that might enable high-throughput, large-area production. However, power conversion efficiencies of these structures have remained low, due in large part to low open-circuit voltages (VOC). Using printing methods, we deposit a layer of colloidal cadmium selenide (CdSe) quantum dots (QDs) onto a wide band-gap organic hole-transporting thin film of N,N'-bis(3-methylphenyl)-N,N'-bis-(phenyl)-9,9-spirobiuorene (spiro-TPD) in order to form a unique planar heterojunction photovoltaic device. This structure is found to produce much higher VOC than previously predicted for donor/acceptor heterojunction photovoltaics. Absorption and charge generation occur primarily in the QD layer and indium tin oxide (ITO) provides the top contact, allowing for exceptional device stability and full transparency below the QD bandgap of 2.0 eV. Overall power conversion efficiencies remain low at 0.03% because only a small percentage of the incident light is absorbed (4% at the rst QD excitonic peak of 2.1 eV) and ll factors are near 0.4, yet VOC is 1.3V. (cont.) The high VOC is remarkable for an architecture with symmetric electrodes and exceeds the offset between the highest occupied molecular orbital (HOMO) of the acceptor (near 5.2 eV) and the lowest unoccupied molecular (LUMO) orbital of the QDs (near 4.6 eV). The internal quantum efficiency (IQE) exhibits a strong dependence on QD lm thickness and reaches a maximum of 30% at a thickness of 3-4 monolayers, indicating that transport losses dominate photocurrent generation for QD thicknesses above 4-5 monolayers. From the bias-dependence of quantum efficiency, we identify an intensity-independent compensation voltage V0 of 1.5 V that represents the maximum attainable VOC. Investigation of the bias-dependence of the photocurrent decay transients identifies charge diffusion as the dominant mechanism responsible for photocurrent generation and reveals a vast discrepancy between the time constant associated with charge extraction (0.6 s, measured at 0V) and that of recombination (0.4 [mu]s, measured at 2 V). An alternative model for VOC is presented that considers the dark current in forward bias as the critical mechanism determining VOC. We conclude that suppression of recombination across the spiro-TPD heterojunction interface forces recombination to occur predominantly in the QD lm. Electroluminescence from the QD layer recombination that hole injection from spiro-TPD into the QD layer and recombination in the QD layer is, in part, responsible for current ow in forward bias. Because the device architecture is straightforward and the fabrication techniques are simple, QD tandem cells are easily attained, furthering the prospect for high conversion efficiencies coupled with the potential for scaleable manufacturability.
Description
Thesis (Ph. D.)--Massachusetts Institute of Technology, Dept. of Electrical Engineering and Computer Science, 2010. This electronic version was submitted by the student author. The certified thesis is available in the Institute Archives and Special Collections. Cataloged from student submitted PDF version of thesis. Includes bibliographical references (p. 227-241).
Date issued
2010Department
Massachusetts Institute of Technology. Department of Electrical Engineering and Computer SciencePublisher
Massachusetts Institute of Technology
Keywords
Electrical Engineering and Computer Science.