Numerical analysis of radio-frequency sheath-plasma interactions in the ion cyclotron range of frequencies
Author(s)
Kohno, Haruhiko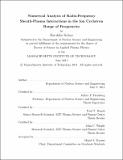
DownloadFull printable version (4.539Mb)
Other Contributors
Massachusetts Institute of Technology. Dept. of Nuclear Science and Engineering.
Advisor
Jerey P. Freidberg.
Terms of use
Metadata
Show full item recordAbstract
Electromagnetic plasma waves in the ion cyclotron range of frequencies (ICRF) are routinely used in magnetic fusion experiments to heat plasmas and drive currents. However, many experiments have revealed that wave energy losses in the plasma edge and at the wall are significant, and detected that the acceleration of ions into the walls due to the formation of radio-frequency (RF) sheaths is one of the root causes of this problem. Since the RF-enhanced sheaths have many undesirable effects, such as impurity production and hot spot generation, a predictive numerical tool is required to quantitatively evaluate these effects with complicated boundary shapes of tokamaks taken into account. In this thesis the numerical code that solves self-consistent RF sheath-plasma interactions in the scrape-off layer for ICRF heating is developed based on a nonlinear finite element technique and is applied to various problems in the one-dimensional (1D) and two-dimensional (2D) domains corresponding to simplified models for the poloidal plane of a tokamak. The present code solves for plasma waves based on the cold plasma model subject to the sheath boundary condition, in which the most important physics that happens in the sheath is captured without using the field quantities in the sheath. Using the developed finite element code, several new properties of the RF sheath plasma interactions are discovered. First, it is found in the 1D domain that multiple roots can be present due to the resonance of the propagating slow wave and its nonlinear interaction with the sheath. Second, sheath-plasma waves are identified in a 2D slab geometry, and it is proved in conjunction with an electrostatic 2D sheath mode analysis that the sheath-plasma wave only appears in the vicinity of the sheath surface if the plasma density is greater than the lower hybrid density, and its wavelength depends on various parameters. Third, as a consequence of the self-consistent interaction between the propagating slow wave and the sheath, it is shown that the electric field distribution pattern in the plasma smoothly varies along the magnetic field lines between the conducting-wall and quasi-insulating limits. In the numerical analysis employing the 2D domain whose scale is equivalent to the Alcator C-Mod device, it is demonstrated that the calculated sheath potential can reach the order of kV, which is sufficient to yield enhanced sputtering at the wall. In addition, it is shown that the sheath potential in the close vicinity of the antenna current strap can be insensitive to the direction of the background magnetic field in the RF sheath dominated regime. Further, it is found from a series of nonlinear calculations that the sheath potential sensitively varies depending on the plasma density and electron temperature, which is consistent with the scaling derived from the Child-Langmuir law and the definition of the RF sheath potential. Lastly, a new finite element approach, which is named the finite element wave-packet method, is developed for the purpose of solving for multiscale plasma waves in the tokamak poloidal plane accurately with reasonable computational cost. This method is established by combining the advantages of the finite element and spectral methods, so that important properties in the finite element method, such as the sparsity of the global matrix and the ease in satisfying the boundary conditions, are retained. The present scheme is applied to some illustrative 1D multiscale problems, and its accuracy improvement is demonstrated through comparisons with the conventional finite element method.
Description
Thesis (Sc. D. in Applied Plasma Physics)--Massachusetts Institute of Technology, Dept. of Nuclear Science and Engineering, 2011. This electronic version was submitted by the student author. The certified thesis is available in the Institute Archives and Special Collections. Cataloged from student submitted PDF version of thesis. Includes bibliographical references (p. 183-191).
Date issued
2011Department
Massachusetts Institute of Technology. Department of Nuclear Science and EngineeringPublisher
Massachusetts Institute of Technology
Keywords
Nuclear Science and Engineering.