A data-driven neuromuscular model of walking and its application to prosthesis control
Author(s)
Markowitz, Jared (Jared John)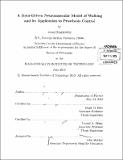
DownloadFull printable version (11.66Mb)
Other Contributors
Massachusetts Institute of Technology. Department of Physics.
Advisor
Hugh M. Herr and Leonid A. Mirny.
Terms of use
Metadata
Show full item recordAbstract
In this thesis we present a data-driven neuromuscular model of human walking and its application to prosthesis control. The model is novel in that it leverages tendon elasticity to more accurately predict the metabolic consumption of walking than conventional models. Paired with a reflex-based neural drive the model has been applied in the control of a robotic ankle-foot prosthesis, producing speed adaptive behavior. Current neuromuscular models significantly overestimate the metabolic demands of walking. We believe this is because they do not adequately consider the role of elasticity; specifically the parameters that govern the force-length relations of tendons in these models are typically taken from published values determined from cadaver studies. To investigate this issue we first collected kinematic, kinetic, electromyographic (EMG), and metabolic data from five subjects walking at six different speeds. The kinematic and kinetic data were used to estimate muscle lengths, muscle moment arms, and joint moments while the EMG data were used to estimate muscle activations. For each subject we performed a kinematically clamped optimization, varying the parameters that govern the force-length curve of each tendon while simultaneously seeking to minimize metabolic cost and maximize agreement with the observed joint moments. We found a family of parameter sets that excel at both objectives, providing agreement with both the collected kinetic and metabolic data. This identification allows us to accurately predict the metabolic cost of walking as well as the force and state of individual muscles, lending insight into the roles and control objectives of different muscles throughout the gait cycle. This optimized muscle-tendon morphology was then applied with an optimized linear reflex architecture in the control of a powered ankle-foot prosthesis. Specifically, the model was fed the robot's angle and state and used to command output torque. Clinical trials were conducted that demonstrated speed adaptive behavior; commanded net work was seen to increase with walking speed. This result supports both the efficacy of the modeling approach and its potential utility in controlling life-like prosthetic limbs.
Description
Thesis (Ph. D.)--Massachusetts Institute of Technology, Dept. of Physics, 2013. Cataloged from PDF version of thesis. Includes bibliographical references (pages 119-123).
Date issued
2013Department
Massachusetts Institute of Technology. Department of PhysicsPublisher
Massachusetts Institute of Technology
Keywords
Physics.