Co-optimizing silicon solar cell processing for efficiency and throughput
Author(s)
Morishige, Ashley E. (Ashley Elizabeth)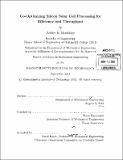
DownloadFull printable version (6.559Mb)
Other Contributors
Massachusetts Institute of Technology. Department of Mechanical Engineering.
Advisor
Tonio Buonassisi.
Terms of use
Metadata
Show full item recordAbstract
Crystalline silicon solar cells are a proven renewable energy technology, but they have yet to reach low costs commensurate with subsidy-free, grid-scale adoption. To achieve the widespread adoption of photovoltaics, the cost per unit of electricity must be reduced by increasing solar cell efficiency. Parts per trillion concentrations of iron impurities in the silicon material can severely limit solar cell efficiency. Iron can be found in both precipitated and point defect form in silicon. Both forms are detrimental to final solar cell efficiency, but their negative impact can be mitigated during solar cell processing. In a standard solar cell process, the phosphorus diffusion step is the key opportunity to redistribute iron impurities because it is the step with the largest thermal budget. Phosphorus diffusion process optimization for solar cell material so far typically consists of one or more isothermal steps followed by a cooling step. Iron silicide precipitates can be dissolved at high temperatures, whereas at lower temperatures, interstitially dissolved iron is driven to the phosphorus-rich layer. Previous optimizations typically maximize minority carrier lifetime without constraining process time and device parameters. This thesis explores a novel phosphorus diffusion process in which there are no isothermal steps. The goal of this work is to demonstrate simultaneous maximization of minority-carrier lifetime, while maintaining high process throughput and steady emitter sheet resistance. Predictive simulation, electrical characterization techniques, and synchrotron-based X-ray fluorescence were combined to compare this new processing approach to standard solar cell processing. This continuously ramped temperature processing may be a promising approach for maximizing solar cell performance, maintaining reasonable manufacturing rates, and achieving a target sheet resistance.
Description
Thesis: S.M., Massachusetts Institute of Technology, Department of Mechanical Engineering, 2013. Cataloged from PDF version of thesis. Includes bibliographical references (pages 67-71).
Date issued
2013Department
Massachusetts Institute of Technology. Department of Mechanical EngineeringPublisher
Massachusetts Institute of Technology
Keywords
Mechanical Engineering.