Silicon field-effect sensors for biomolecular assays
Author(s)
Cooper, Emily Barbara, 1977-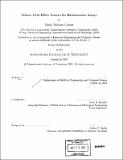
DownloadFull printable version (10.84Mb)
Other Contributors
Massachusetts Institute of Technology. Department of Electrical Engineering and Computer Science.
Advisor
Scott R. Manalis.
Terms of use
Metadata
Show full item recordAbstract
System-level understanding of biological processes requires the development of novel biosensors capable of quantitative, real-time readout of molecular interactions. Label-free detection methods can minimize costs in time and resources by obviating preparatory steps necessary with label-based methods. They may further be valuable for monitoring biomolecular systems which are difficult or impossible to tag, or for which reporter molecules interfere with biological function. Field-effect sensing is a method of directly sensing intrinsic electrical charge associated with biomolecules without the need for reporter molecules. Microfabrication of field-effect biosensors enables their integration in compact microanalytical systems, as well as the potential to be scaled down in size and up in number. Applying field-effect sensing to the detection and real-time monitoring of specific molecular interactions has long been of interest for protein and nucleic acids analysis. However, these applications are inhibited by serious practical limitations imposed by charge screening in solution. The development of effective measurement techniques requires inquiry into aspects of device engineering, surface chemistry, and buffer conditions. This thesis describes a body of experimental work that investigates the feasibility of label-free analysis of biomolecular interactions by field-effect. This work begins with the microfabrication of field-effect sensors with extremely thin gate oxide, which enables improved surface potential resolution over previously reported sensors. (cont.) The performance of these sensors has been characterized in terms of drift, noise, and leakage. To better understand the applicability of these sensors, we have characterized the sensors' response to pH, adsorption of polyelectrolyte multilayers, and high-affinity molecular recognition over a range of buffer conditions. Direct, label-free detection of DNA hybridization was accomplished by combining the high-resolution sensors, with enabling surface chemistry, and a differential readout technique. Finally, we explore the lateral scaling limits of potentiometry by applying a novel nanolithographic technique to the fabrication of a single electron transistor that demonstrates Coulomb oscillations at room temperature.
Description
Thesis (Ph. D.)--Massachusetts Institute of Technology, Dept. of Electrical Engineering and Computer Science, 2003. Includes bibliographical references.
Date issued
2003Department
Massachusetts Institute of Technology. Department of Electrical Engineering and Computer SciencePublisher
Massachusetts Institute of Technology
Keywords
Electrical Engineering and Computer Science.