Amphiphilic gold nanoparticles: mechanisms for interaction with membranes and applications in drug and vaccine delivery
Author(s)
Atukorale, Prabhani U. (Prabhani Upeka)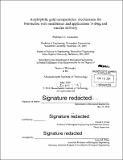
DownloadFull printable version (12.17Mb)
Other Contributors
Massachusetts Institute of Technology. Department of Biological Engineering.
Advisor
Darrell J. Irvine.
Terms of use
Metadata
Show full item recordAbstract
Materials that can interact with and transit membranes without toxic bilayer disruption or poration are of great interest in the drug delivery field. These materials can presumably bypass endocytosis to directly enter the cell cytosol through the plasma membrane, which is often the desired site of action for therapeutics, thereby avoiding potential and likely cargo degradation if trapped in endosomes. Alternatively, if these materials are endocytosed, they are often able to escape the endosome by interacting with and transiting the endosomal membrane, and moving into the cytosol. Here, we present a unique membrane-interacting, amphiphilic gold nanoparticle (amph-AuNP) system, which we show can interact with, embed within, and even penetrate through multiple adjacent lipid bilayers without evidence of membrane disruption or poration. By virtue of these key properties, we also present these amph-AuNPs as effective carriers for therapeutic molecules. Using a one-step reaction, we synthesized small -2-4 nm core size amph- AuNPs with an amphiphilic ligand shell comprised of one or two alkanethiols. Each amph-AuNP is coated by a mixture of long-chain mercaptoundecanesulfonate (MUS) terminated by a water-soluble sulfonate group, and in some cases, short-chain hydrophobic octanethiol (OT). First, we describe our efforts to adapt and develop a method to synthesize giant multilamellar model membranes to study amph-NP-membrane interaction in a well-defined setting. We show that giant membranes can be synthesized and fine-tuned by varying lipid composition and buffer salt concentration, can be fluorescently labeled with lipid tracers, and can be analyzed robustly with confocal microscopy and flow cytometry. Second, we describe our systematic analysis of amph- AuNP and membrane characteristics that influence mechanisms of NP association with bilayers. We study effects of general membrane properties such as electrostatics and phase that govern NP-membrane interactions, and found that NP penetration of bilayers was blocked under conditions where strong electrostatic repulsion or gel-phase lipids were employed. We further studied effects of AuNP core diameter, surface charge, and surface hydrophobicity on NP-membrane interactions at the nanoscale. We found that MUS particles with an optimal gold core size -2- 3nm in diameter and MUS:OT particles of a broader size range were capable of inducing hemifusion between liposomal membranes, while MUS:OT 2:1 particles of intermediate hydrophobicity were capable of spontaneously aggregating within the bilayer of vesicles to form Janus egg-like morphologies. Third, we built on these NP membrane-embedding properties to explore and characterize NP-embedding in erythrocyte membranes, with particular attention to the glycocalyx and membrane fluidity, for the future application of constructing therapeutic erythrocyte 'pharmacytes' in situ. Finally, we describe work in engineering amph-AuNPs to carry short antigenic peptide cargoes for in vivo vaccine applications, where immunization experiments have shown much promise for antigen-ferrying amph-AuNPs in eliciting robust and long-lasting CD8+ T cell responses.
Description
Thesis: Ph. D., Massachusetts Institute of Technology, Department of Biological Engineering, 2014. Cataloged from PDF version of thesis. Includes bibliographical references (pages 70-82).
Date issued
2014Department
Massachusetts Institute of Technology. Department of Biological EngineeringPublisher
Massachusetts Institute of Technology
Keywords
Biological Engineering.