Electrostatic charging of jumping droplets on superhydrophobic nanostructured surfaces : fundamental study and applications
Author(s)
Preston, Daniel J. (Daniel John)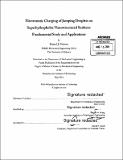
DownloadFull printable version (13.55Mb)
Other Contributors
Massachusetts Institute of Technology. Department of Mechanical Engineering.
Advisor
Evelyn N. Wang.
Terms of use
Metadata
Show full item recordAbstract
Condensation is a ubiquitous process often observed in nature and harnessed in many industrial processes such as power generation, desalination, thermal management, and building environmental control. Recent advancements in surface engineering have offered new opportunities to enhance condensation heat transfer by drastically changing the wetting properties of the condenser surface. Specifically, the development of superhydrophobic surfaces has been pursued to enhance condensation heat transfer, where the low droplet surface adhesion and small droplet departure sizes increase the condensation heat transfer coefficient. Specifically, when two or more small (~10-100 [mu]m) droplets coalesce on a superhydrophobic surface, they can spontaneously jump away from the surface due to the reduced droplet-surface adhesion and release of excess surface energy, which has been shown to increase heat transfer by 30 - 40% compared to that observed during gravitational shedding of droplets. While this droplet jumping phenomenon has been studied on a range of surfaces, past work has neglected electrostatic interactions and assumed charge neutrality of the droplets. Here, we show that jumping droplets on a variety of superhydrophobic surfaces, including copper oxide, zinc oxide, and silicon nanopillars, gain a net positive charge that causes them to repel each other mid-flight. The charge is determined experimentally by observing droplet motion in a uniform electric field. The mechanism for the charge accumulation is associated with the formation of the electric double layer at the droplet-coating interface and subsequent charge separation during droplet jumping governed by the fast time scales of droplet coalescence. One application of this charging phenomenon is further enhancement of condensation heat transfer by preventing droplet reversal and return to the condenser surface due to the presence of vapor flow towards the surface, which increases the drag on the jumping droplets. This effect limits the possible heat transfer enhancement because larger droplets form upon droplet return to the surface that impede heat transfer until they can be either removed by jumping again or finally shedding via gravity. By characterizing individual droplet trajectories during condensation on superhydrophobic nanostructured copper oxide surfaces, this vapor flow entrainment is shown to dominate droplet motion for droplets smaller than R ~ 30 [mu]m at moderate heat fluxes (q" > 2 W/cm2 ). Subsequently, electric-field-enhanced (EFE) condensation is demonstrated, whereby an externally applied electric field prevents jumping droplet return due to the positive charge obtained by the droplets upon jumping. As a result, with scalable superhydrophobic CuO surfaces, a 50% higher overall condensation heat transfer coefficient is demonstrated compared to a jumping-droplet surface with no applied field for low supersaturations (<1.12). Another application of charged jumping droplets is use of these droplets for electrostatic energy harvesting. Here, the charged droplets jump between superhydrophobic copper oxide and hydrophilic copper surfaces to create an electrostatic potential and generate power during formation of dew under atmospheric conditions. Power densities of ~0.06 nW/cm 2 are demonstrated, which, in the near term, can be improved to ~1 [mu]W/cm 2 . This work demonstrates a surface engineered platform that is low cost and scalable for atmospheric energy harvesting and electric power generation. These applications of charged jumping droplets offer new avenues for improving the performance of self-cleaning and anti-icing surfaces as well as thermal diodes, and may also provide a competitive mode of energy harvesting from temperature gradients.
Description
Thesis: S.M., Massachusetts Institute of Technology, Department of Mechanical Engineering, 2014. Cataloged from PDF version of thesis. Includes bibliographical references (pages 65-71).
Date issued
2014Department
Massachusetts Institute of Technology. Department of Mechanical EngineeringPublisher
Massachusetts Institute of Technology
Keywords
Mechanical Engineering.