Mechanisms governing the growth, reactivity and stability of iron sulfides
Author(s)
Herbert, Francis William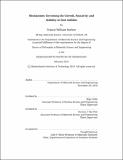
DownloadFull printable version (10.43Mb)
Other Contributors
Massachusetts Institute of Technology. Department of Materials Science and Engineering.
Advisor
Bilge Yildiz and Krystyn J. Van Vliet.
Terms of use
Metadata
Show full item recordAbstract
The kinetics of electrochemical processes in ionic materials are fundamentally governed by dynamic events at the atomic scale, including point defect formation and migration, and molecular interactions at the surface. A corrosion system comprising an iron sulfide film (passive layer) formed on iron or steel in contact with an hydrogen sulfide (H₂S)- rich fluid can thus, in principle, be modeled by a series of unit reaction steps that control the rate of degradation under given thermodynamic conditions. This overarching thesis goal necessitates a concerted experimental and computational approach to determine the relevant kinetic parameters such as activation barriers Ea and rate constants v₀ for the homogeneous and heterogeneous reactions of interest. These fundamental values can be obtained experimentally via temperature-dependent measurements on pure, model iron sulfide samples. This thesis therefore consists of three case studies on the stable Fe-S phases pyrrhotite (Fe₁-xS) and pyrite (FeS₂) to identify the elementary corrosion mechanisms and their kinetic parameters. Pyrrhotite is of interest because the off-stoichiometry of this phase leads to relatively rapid bulk processes such as diffusion; pyrite has a comparitively inert bulk but this work showed that it has a chemically labile surface. The first study focuses on two basic, rate-controlling steps in the growth of pyrrhotite: cation diffusion and sulfur exchange at the surface. First, iron self-diffusivity *DFe is determined across the temperature range 170-400 °C through magnetokinetic studies of the diffusion-driven "[lambda]" magnetic transformation, as well as direct tracer diffusion measurements in Fe₁-xS crystals using secondary ion mass spectrometry (SIMS). This range encompasses the sponteneous magnetic and structural order-disorder temperature TN = 315 °C in pyrrhotite. The effect of spontaneous magnetization below TN is to increase the Fe vacancy migration energy by a combined 40% increasing Ea for diffusion from 0.83 eV in paramagnetic Fe₁-xS to ~1.20 eV in the fully magnetized state. An extrapolation of the Arrhenius law from the paramagnetic regime would therefore overestimate actual diffusivities by up to 10² times at 150 °C. Second, the surface exchange of sulfur from H₂S into the solid state in Fe₁-xS is measured using electrical conductivity relaxation, yielding Ea = 1.1 eV for sulfur incorporation into pyrrhotite. With their similar thermal dependence, there is no clear temperature crossover from cation diffusion- to surface exchange-limiting regimes, or vice versa. Instead, surface exchange is expected to constrain pyrrhotite growth for films under ~100 [mu]m thickness, beyond which diffusion becomes the rate limiting mechanism, independent of external driving factors such as temperature. The second study explores the role of surface electronic states on the electrochemical reactivity of pyrite. Charge transfer between a solid surface and an adsorbate such as H₂S requires the mutual availability of filled/empty electronic states at the same energy level. The semiconducting FeS₂(100) surface is predicted to have intrinsic surface states (SS's) from Fe and S dangling bonds, as well as extrinsic SS's related to delocalized defects at the surface, both of which would affect charge transfer characteristics. A novel, broadly-applicable methodology is developed in this thesis to quantify the energy and density of these SS's, based on experimental scanning tunneling microscopy / spectroscopy (STM/STS) in conjunction with first principles tunneling current modeling. As a result, a decreased surface band gap Eg of 0.4 eV, compared to 0.95 eV in bulk pyrite, is measured. The findings highlight the need to differentiate between bulk and surface electronic structure when assessing heterogeneous reactivity, and have implications for the use of FeS₂ in potential technological applications, for example as a photovoltaic adsorber. Finally, the dynamics of point defect formation and clustering on FeS₂(100) under high-temperature, reducing conditions are investigated to understand the stability of the surface under extreme conditions. Synchrotron x-ray photoelectron spectroscopy (XPS) is used to measure a formation energy [delta]Hf for sulfur vacancies in the topmost atomic layer of 0.1 eV up to approximately 240 °C. Above this temperature, however, point defects are shown to condense into surface pits as measured by scnaning tunneling microscopy (STM). The combined, experimental XPS and STM results are replicated with high precision by a kinetic Monte Carlo (kMC) simulation, developed by Aravind Krishnamoorthy towards his doctoral thesis, of surface degradation on realistic length-and timescales of 10-¹⁰ - 10-⁷ m and up to several hours, respectively. The findings have implications for the initiation of surface breakdown via pitting in ionic passive films, as well as providing a broader understanding of the non-stoichiometry of the pyrite surface. The common thread is a focus on events at the atomic and electronic scale, with an emphasis on point defects. The results thereby facilitate a bottom-up approach to modeling electrochemical processes such as corrosion in Fe-S phases, in which the unit steps are cast into probabilistic simulation tools. While the three studies here comprise only a partial examination of the atomic-scale events regulating the behavior of Fe-S passive layers, this approach makes inroads towards more accurate component lifetime prediction and the design of robust materials for aggressive environments. Moreover, the fundamental surface and bulk physical chemistry of iron sulfides explored in this work has implications beyond corrosion to other uses of these materials, including potential magnetic devices (Fe₁-xS) and earth-abundant photovoltaic and photoelectrochemical adsorbers (FeS₂).
Description
Thesis: Ph. D., Massachusetts Institute of Technology, Department of Materials Science and Engineering, 2015. This electronic version was submitted by the student author. The certified thesis is available in the Institute Archives and Special Collections. Cataloged from student-submitted PDF version of thesis. Includes bibliographical references (pages 135-140).
Date issued
2015Department
Massachusetts Institute of Technology. Department of Materials Science and EngineeringPublisher
Massachusetts Institute of Technology
Keywords
Materials Science and Engineering.