Theoretical and experimental study of solid oxide fuel cell (SOFC) using impedance spectra
Author(s)
Fu, Yeqing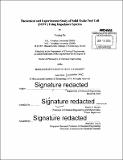
DownloadFull printable version (9.139Mb)
Other Contributors
Massachusetts Institute of Technology. Department of Chemical Engineering.
Advisor
Martin Z. Bazant.
Terms of use
Metadata
Show full item recordAbstract
Solid oxide fuel cell (SOFC) is a promising alternative energy source, with its advantages of high operating efficiency, fuel flexibility, low emissions and relatively low cost. However, there are several challenges concerning the SOFC research. Little is known about the complex interfacial electrochemistry and thermochemistry, and it is also difficult to diagnose problems and optimize cell performance. Therefore, physics-based models are needed to better understand the underlying mechanisms of SOFCs. This research work addressed two important aspects of the numerical modeling of SOFCs: the multicomponent gas diffusion in porous electrode at the anode and the heterogeneous electrocatalysis of oxygen reduction reaction (ORR) at the cathode. First, anode was diagnosed to be mainly controlled by multicomponent gas diffusion inside the anode bulk (supporting) layer, and the Dusty Gas model is identified as an appropriate model to describe the gas diffusion resistance extracted from no bias AC impedance. Anode-supported SOFCs with Ni-yttria-stabilized zirconia (YSZ) anode were used to study the multicomponent gas transport in porous electrodes. A fuel gas mixture of H₂-H₂O-N₂ was fed to the anode and AC impedance data were measured at 800°C by varying hydrogen partial pressure at both no bias and a current of 300 mA. Impedance data were also collected at no bias at three different temperatures (800°C, 850°C and 900°C). For the first time, three models were used to analytically derive the diffusion resistance (Rb), which was then compared to the values extracted from experimental impedance data. The Dusty Gas model yields the best predictions and the tortuosity values derived from Dusty Gas model are found to be independent of feeding gas composition, operating current and temperatures, which is consistent with the fundamental or underlying physics. Moreover, with the anode porosity known to be approximately 46%, the tortuosity derived from the Dusty Gas model is 2.3~3.3, which matches both theoretical expectations and experimental measurements. This gas diffusion resistance analysis using AC impedance greatly improves the way to study the multicomponent gas diffusion within porous electrodes. Secondly, electrocatalysis at the SOFC cathode was studied using symmetric cathode cells, whose no bias AC impedance was investigated and modeled using a physics-based electrocatalysis model, describing the coupled dissociative adsorption of oxygen molecules onto the catalytic lanthanum strontium manganite (LSM) particles and surface diffusion of adsorbed species, assuming the charge transfer reaction is relatively fast and at equilibrium. A Gerischer type impedance response with a reflecting boundary condition was theoretically derived assuming the oxygen adsorption follows Langmuir type kinetics. This cathode electrocatalysis model not only captures the frequency dependence of the no bias AC impedance, it also well represents the oxygen partial pressure (pO₂) dependence. Four different impedance curves at pO₂ 2 of 21%, 15%, 10% and 5% were fitted at the same time, and the model was able to well describe them using one set of physically meaningful fitting parameters. Microstructure of the cathode functional layer (CFL) was also studied using this electrocatalysis model. It was found that the diffusion length Ls, is a critical parameter, whose ratio with respect to the characteristic boundary layer length l[delta], (the Thiele modulus) critically controls the effectiveness of the catalytic activity of the cathode functional layer. These understandings of the anode gas diffusion and cathode electrocatalytic process was used to propose an equivalent circuit for the full solid oxide fuel cell, which captures all important resistances in the SOFC, but is still as simple as possible, in order to minimize the number of fitting parameters. This full cell model greatly helps to break down the AC impedance which has overlapped responses from several processes. The analysis identified the rate limiting step of the full Saint-Gobain button cell to be the cathode electrocatalytic process, which indicates that in order to improve the cell performance, research should be focused on improving the cathode functional layer, by either improving the surface catalytic activity of the LSM particles, or changing the microstructure of the cathode functional layer.
Description
Thesis: Ph. D., Massachusetts Institute of Technology, Department of Chemical Engineering, September 2014. Cataloged from PDF version of thesis. "June 2014." Includes bibliographical references (pages 100-107).
Date issued
2014Department
Massachusetts Institute of Technology. Department of Chemical EngineeringPublisher
Massachusetts Institute of Technology
Keywords
Chemical Engineering.