Simulation and control of dynamic directed self-assembly of nanostructures
Author(s)
Ramaswamy, Sivaraman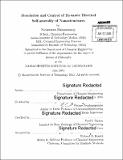
DownloadFull printable version (16.20Mb)
Other Contributors
Massachusetts Institute of Technology. Department of Chemical Engineering.
Advisor
George Stephanopoulos and Paul I. Barton.
Terms of use
Metadata
Show full item recordAbstract
Self-assembled nanoscale structures are the basis for various technological advancements in functional materials, sensors, and molecular circuits and factories. With significant progress in self-assembly of periodic nanostructures (such as monolayers), the focus is now shifting towards non-periodic structures. Control of various interaction force fields (electrostatic, Van der Waals, etc.) between the nanoparticles and external controls can result in the formation of nanostructures with desired geometry. The aim is to design the nanoparticles and the external actuators such that the desired structure can be self-assembled rapidly with high reliability and avoiding any kinetic trapping that an ill-designed energy landscape might cause. Deterministic dynamic modeling of such self-assembled nanostructures, directed by external fields, through a Master Equation approach, leads to a set of differential equations of such large size that even the most efficient solution algorithms are overwhelmed. Thus, model reduction is a key necessity. This thesis presents a methodological approach and specific algorithms, which generate time-varying, reduced-order models for the description of directed self-assembly of nanoparticles by external fields. The approach is based on Finite State Projection and is adaptive, i.e., it generates reduced-order models that vary over time. The algorithm uses event-detection concepts to determine automatically, during simulation, suitable time points at which the projection space and thus the structure of the reduced-order model change, in such a way that the computational load remains low while the upper bound on the simulation error, resulting from model reduction, is lower than a prescribed maximum limit. The thesis also presents an optimal control strategy that can guide any initial random configuration of nanoparticles to a final structure of desired geometry, in minimum time. It employs a multi-resolution view of the dynamically evolving configurations of nanoparticles, which are described through the simulation methodology described before. External charges, attracting or repelling the nanoparticles, are the controls, whose location and intensity are determined by the optimality conditions of the optimal control strategy. To ensure analytic consistency of the parametric sensitivities, during the computation of the optimal controls, and thus guarantee the optimality of the resulting control policy, a priori determination of enlarged constant projection spaces is shown to be essential. The thesis also presents a series of case studies, which illustrate how the proposed methods can be used to simulate effectively directed self-assembly of an appreciable number of nanoparticles, and reach the desired geometry. These case studies also illuminate several of its features, such as: superiority over a static optimal solution; evasion of kinetic traps; and effective handling of combinatorial complications arising for systems with large-size domains and many particles.
Description
Thesis: Ph. D., Massachusetts Institute of Technology, Department of Chemical Engineering, 2015. Cataloged from PDF version of thesis. Includes bibliographical references (pages 111-117).
Date issued
2015Department
Massachusetts Institute of Technology. Department of Chemical EngineeringPublisher
Massachusetts Institute of Technology
Keywords
Chemical Engineering.