Anisotropic dewetting in ultra-thin single-crystal silicon-on-insulator films
Author(s)
Danielson, David T. (David Thomas)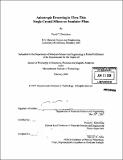
DownloadFull printable version (44.13Mb)
Other Contributors
Massachusetts Institute of Technology. Dept. of Materials Science and Engineering.
Advisor
Lionel C. Kimerling.
Terms of use
Metadata
Show full item recordAbstract
The single crystal silicon-on-insulator thin film materials system represents both an ideal model system for the study of anisotropic thin film dewetting as well as a technologically important system for the development of the next generation of MOSFET devices. The scientific community has misinterpreted the mechanism behind SOI dewetting, attributing it to a stress-driven instability. In this work, we characterize and measure the kinetics and geometry of thermally-activated dewetting in ultra-thin SOI films as a function of SOI thickness, crystallographic patterned-edge orientation, and temperature. Using experimental data and physical reasoning, we strongly argue against the previously existing scientific consensus that SOI dewetting is a stress-driven instability and present a 5-step isotropic surface-energy-driven dewetting model based upon the capillary film edge instability and the generalized Rayleigh instability, in which we integrate existing theories and in addition develop an expression for the edge retraction distance during the initial uniform edge thickening and retraction phase of dewetting at a patterned mesa edge for the first time, predicting a square root dependence with time and an inverse power dependence on film thickness. In our study, we observe and quantify the kinetics of the initial edge retraction dewetting mechanism experimentally for the first time and confirm the square root time dependence and inverse power law film thickness dependence predicted by our new model. We also quantify the edge dewetting front propagation velocity in SOI and determine that it depends on the negative third power of the film thickness, agreeing with the prediction of Jiran and Thompson and with our model. (cont.) We also extract an activation energy for the edge dewetting front propagation velocity of 2.9+-0.3eV, in reasonable agreement with reported values for Si surface diffusion on (111) planes. Furthermore, we present for the first time an anisotropic model for void finger propagation that is able to accurately explain the detailed anisotropic dewetting geometries observed in SOI dewetting. A technique for stabilizing ultra-thin SOI films, dielectric layer edge coverage, is also demonstrated that may prove useful to enable the stabilization of ultra-thin SOI films during fully-depleted SOI transistor fabrication. We also report on the effect of Ge coverage on SOI dewetting kinetics.
Description
Thesis (Ph. D.)--Massachusetts Institute of Technology, Dept. of Materials Science and Engineering, 2008. Includes bibliographical references (p. 153-156).
Date issued
2008Department
Massachusetts Institute of Technology. Department of Materials Science and EngineeringPublisher
Massachusetts Institute of Technology
Keywords
Materials Science and Engineering.