Study of ultranarrow superconducting NbN nanowires and nanowires under strong magnetic field for photon detection
Author(s)
Herder, Charles H. (Charles Henry), III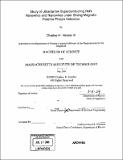
DownloadFull printable version (11.88Mb)
Other Contributors
Massachusetts Institute of Technology. Dept. of Physics.
Advisor
Karl K. Berggren.
Terms of use
Metadata
Show full item recordAbstract
Photon detection is an integral part of experimental physics, high-speed communication, as well as many other high-tech disciplines. In the realm of communication, unmanned spacecraft are travelling extreme distances, and ground stations need more and more sensitive and selective detectors to maintain a reasonable data rate. In the realm of computing, some of the most promising new forms of quantum computing require consistent and efficient optical detection of single entangled photons. Due to projects like these, demands are increasing for ever more efficient detectors with higher count rates. The Superconducting Nanowire Single-Photon Detector (SNSPD) is one of the most promising new technologies in this field, being capable of counting photons as faster than 100MHz and with efficiencies around 50%. Currently, the leading competition is from the geiger-mode avalanche photodiode, which is capable of ~20 ~70% efficiency at a ~5MHz count rate depending on photon energy. In spite of this, the SNSPD is still a brand-new technology with many potential avenues unexplored. Therefore, it is still possible that we can achieve even better efficiencies and count rates to keep up with the requirements of burgeoning technologies. This photon detector consists of a meandering superconducting nanowire biased close to its critical current. In this regime, a single incident photon can cause a section of the detector to switch to normal conduction, producing a voltage pulse due to its now-finite resistance. An electron micrograph is given in figure 1. The intrinsic limitations of the detector (disregarding the optical coupling mechanism and the support electronics) are dominated by two primary points. First is the efficiency with which the detector converts an absorbed photon into a voltage pulse. This is controlled by the behavior of the excited electrons at the point of incidence. I will discuss this in greater detail in the next section. The second is the electrothermal time constant of the detector. This limits the relaxation time of the detector and therefore limits the maximum rate at which the detector can count photons. As we will see, detection efficiency increases as the number of Cooper pairs that need to be excited into the normal state to switch conduction modes decreases. One way to decrease the bandgap is to decrease the cross-section of the wire. This has already been shown to increase detection efficiency, but this cannot be done to arbitrarily narrow wires. Not only is there a limitation to fabrication, but there are also interesting quantum effects that occur at very narrow wire widths. Note that much of the research that has been done to understand these quantum effects has been undertaken on wires much wider than those we will be using. Simultaneously, most of the materials used previously have coherence lengths much longer than NbN. Therefore, even though our wires are narrower by a substantial factor, they are still wider than the coherence length of NbN. As such the validity of the one-dimensional approximation to be presented in in 2.2 is debatable for our wires. However, it should be apparent that regardless of behavior, thermal and quantum phase slips will be one of the limiting factors in producing ultra-narrow nanowire photon detectors. Until now, photon detectors have only used current biasing techniques. However, it is well known that both magnetic field and current have the effect of reducing the energy required to excite superconducting charge carriers. Therefore, it may be possible to detect photons using magnetic field close to H, instead of current close to Ic. It is important to note, however, that the readout of the detector in its current configuration depends on some bias current to produce a voltage pulse. Therefore, with the current detector architecture, one still needs a significant bias current. For my thesis, I have first investigated the theory of supercurrents in ultranarrow wires and confirmed the behavior of this theory with our materials and fabrication techniques in order to establish a lower bound for wire width where photon detection is still possible. In addition, I have constructed and executed an initial experiment to test how photon detectors behave under magnetic field bias conditions. I have measured how these different bias conditions affect the efficiency of the detector as well as the dark count rate.
Description
Thesis (S.B.)--Massachusetts Institute of Technology, Dept. of Physics, 2009. Includes bibliographical references (leaves 32-34).
Date issued
2009Department
Massachusetts Institute of Technology. Department of PhysicsPublisher
Massachusetts Institute of Technology
Keywords
Physics.