Role of cavitation, surfactants, and their synergism in transdermal sonophoresis
Author(s)
Polat, Bariș E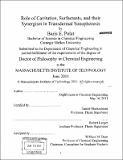
DownloadFull printable version (24.00Mb)
Other Contributors
Massachusetts Institute of Technology. Dept. of Chemical Engineering.
Advisor
Daniel Blankschtein and Robert Langer.
Terms of use
Metadata
Show full item recordAbstract
The research described in this thesis represents a significant advancement in the current mechanistic understanding of low-frequency ultrasound-mediated transdermal drug delivery. Specifically, while prior research has focused on a more general understanding of the mechanisms associated with low-frequency sonophoresis (LFS), this thesis directly investigates the mechanisms associated with phenomena that occur due to LFS skin treatment. These include a deeper understanding of: i) the reasons for heterogeneous perturbation of skin treated with LFS, ii) the physical mechanism that causes synergism between LFS and chemical penetration enhancers (CPEs), iii) the interactions between traditional and non-traditional surfactants in LFS skin treatments, and iv) the effects of LFS and LFS/CPEs on skin structural perturbation. In the first study of this thesis, the intrinsic properties and formation mechanisms of localized-transport regions (LTRs), and the surrounding less-perturbed non-LTRs, of lowfrequency sonophoresis-treated skin are investigated. By independently analyzing LTR, non- LTR, and total skin samples treated at multiple LFS frequencies, it was found that the pore radii (rpore) within non-LTRs are frequency-independent, ranging from 18.2 - 18.5 A, but significantly larger than rpore of native skin samples (13.6 A). Conversely, rpore within LTRs increases significantly with decreasing frequency from 161 A, to 276 A, and to ao (>300 A) for LFS/SLStreated skin at 60 kHz, 40 kHz, and 20 kHz, respectively. These findings suggest that different mechanisms contribute to skin permeability enhancement within each skin region. Therefore, it was proposed that the enhancement mechanism within LTRs is the frequency-dependent process of cavitation-induced microjet collapse at the skin surface, while the increased rpore values in non-LTRs are likely due to SLS perturbation, with enhanced penetration of SLS into the skin resulting from the frequency-independent process of acoustic streaming. Next, the effects of a non-traditional surfactant on LFS treatment were analyzed through a case study with the commonly used fluorescent dye sulforhodamine B (SRB). SRB is often considered to be a purely hydrophilic molecule, having no impact on bulk or interfacial properties of aqueous solutions. However, it was demonstrated that SRB is in fact an amphiphile, with the ability to adsorb at an air/water interface and to incorporate into sodium lauryl sulfate (SLS) micelles. In fact, SRB reduced the surface tension of water by up to 23 mN/m, and the addition of SRB to an aqueous SLS solution was found to induce a significant decrease in the critical micelle concentration (cmc) of SLS. Molecular dynamics revealed that SRB has defined polar "head" and non-polar "tail" regions when adsorbed at the air/water interface as a monomer. These findings have significant implications into LFS-mediated transdermal drug delivery, as the inclusion of SRB into an LFS coupling solution can cause a synergistic increase in skin permeability enhancement. However, in the presence of SLS, SRB actually causes an antagonistic effect due to the resulting change in bulk and interfacial properties. (cont.) The next investigation of this thesis focused on understanding the synergism between LFS and CPEs (e.g., surfactants). In spite of identifying that the origin of this synergism is the increased penetration and subsequent dispersion of CPEs in the skin in response to LFS treatment, no prior study had proposed a mechanism to explain how LFS induces the observed increased transport of CPEs. In this study, a physical mechanism by which the transport of all CPEs is expected to have significantly increased flux into the localized-transport regions (LTRs) of LFS-treated skin was proposed. Specifically, the collapse of acoustic cavitation microjets within LTRs was shown to induce a convective flux. In addition, because amphiphilic molecules are able to adsorb onto the gas/water interface of cavitation bubbles, amphiphiles were shown to have an additional adsorptive flux. In this sense, the cavitation bubbles were shown to effectively act as carriers for amphiphilic molecules, delivering surfactants directly into the skin when they collapse at the skin surface as cavitation microjets. The flux equations derived for the LTRs and non-LTRs of LFS-treated skin, compared to that for untreated skin, explained how the transport of all CPEs, and to an even greater extent amphiphilic CPEs, increases during LFS treatment. The flux model was supported with experiments involving a non-amphiphilic CPE (propylene glycol) and both nonionic and ionic amphiphilic CPEs (octyl glucoside and SLS), by measuring the flux of each CPE into untreated skin and the LTRs and non-LTRs of LFS-treated skin. Data showed excellent agreement with the expected trends from the flux model. The final study of this thesis investigated the effect of SLS on skin structural perturbation when utilized simultaneously with LFS. Pig full-thickness skin (FTS) and pig split-thickness skin (STS) treated with LFS/SLS and LFS were analyzed in the context of the aqueous porous pathway model to quantify skin perturbation through changes in skin pore radius and porosity-totortuosity ratio (e/t). In addition, skin treatment times required to attain specific levels of skin electrical resistivity were analyzed to draw conclusions about the effect of SLS on reproducibility and predictability of skin perturbation. It was found that LFS/SLS-treated FTS, LFS/SLS-treated STS, and LFS-treated FTS exhibited similar skin perturbation. However, LFStreated STS exhibited significantly higher skin perturbation, suggesting greater structural changes to the less robust STS induced by the purely physical enhancement mechanism of LFS. Evaluation of c/c values revealed that LFS/SLS-treated FTS and STS have similar transport pathways, while LFS-treated FTS and STS have lower cF/ values. In addition, LFS/SLS treatment times were much shorter than LFS treatment times for both FTS and STS. Moreover, the simultaneous use of SLS and LFS not only results in synergistic enhancement, as reflected in the shorter skin treatment times, but also in more predictable and reproducible skin perturbation. In conclusion, the research conducted in this thesis has contributed to the advancement of the molecular and cellular-level understanding of phenomena associated with LFS-mediated transdermal skin permeability enhancement. Additionally, the insights provided by this thesis could lead to the development of optimized LFS treatment protocols and improved LFS coupling solution formulations. This would permit attaining the desired skin permeability using milder LFS treatment conditions and smaller skin treatment areas. This could also lead to lower power requirements of LFS devices, thereby leading to miniaturization of devices and the creation of more commercially-viable LFS equipment.
Description
Thesis (Ph. D.)--Massachusetts Institute of Technology, Dept. of Chemical Engineering, 2011. Cataloged from PDF version of thesis. Includes bibliographical references.
Date issued
2011Department
Massachusetts Institute of Technology. Department of Chemical EngineeringPublisher
Massachusetts Institute of Technology
Keywords
Chemical Engineering.