Towards first-principles electrochemistry
Author(s)
Dabo, Ismaila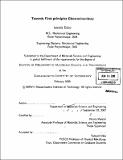
DownloadFull printable version (35.48Mb)
Other Contributors
Massachusetts Institute of Technology. Dept. of Materials Science and Engineering.
Advisor
Nicola Marzari.
Terms of use
Metadata
Show full item recordAbstract
This doctoral dissertation presents a comprehensive computational approach to describe quantum mechanical systems embedded in complex ionic media, primarily focusing on the first-principles representation of catalytic electrodes under electrochemical conditions. The accurate electrostatic description of electrified metal-solution interfaces represents a persistent challenge for ab-initio simulations and an essential requisite for predicting the electrical response of electrochemical convertors-i.e., the correspondence between the macroscopic voltage and the microscopic interfacial charge distribution. The approach consists of controlling the electrode voltage via its conjugate extensive variable, namely, the charge of the system. As a preliminary to the study of electrified interfaces in ionic media, we analyze charged slabs in vacuum subject to periodic boundary conditions. We show that the corrective potential (defined as the difference between the exact open-boundary potential and the periodic potential obtained from a Fourier transform) varies smoothly over space, allowing for its determination on a coarse mesh using optimized electrostatic solvers. Because this scheme takes into account exact open boundary conditions, its performance is considerably superior to that of conventional corrective methods. Extending this computational scheme, we present an efficient approach to model electrochemical systems under realistic conditions, based on a first-principles description of the interface region and on a continuum representation of the ionic solvent. (cont.) We demonstrate that the ionicsolution contribution to the electrostatic potential-the ionic solvent reaction field--can be computed independently at low cost simultaneously using fast Fourier transforms and multigrid techniques, and highlight the importance of adopting adequate electrochemical boundary conditions to correctly predict the electrical response of electrode-electrolyte interfaces. In order to probe and validate the electrochemical model, we study the vibrational Stark effect-i.e., the influence of the applied voltage on the vibrational properties-for carbon monoxide adsorbed on transition metal surfaces, a phenomenon whose description requires an accurate representation of the interfacial electric field. We start out the analysis by examining the vibrational properties of CO adsorbed on clean and ruthenium-covered platinum substrates. The calculated C-O stretching frequencies are found to be in excellent agreement with experimental measurements despite the frequent qualitative failures of local and semilocal exchange-correlation functionals in predicting adsorption energies for CO on transition metals. We then introduce an orbital-resolved force analysis to clarify the electronic origins of the C-O red shifts, and present a sensitivity analysis to assess the influence the HOMO and LUMO hybridizations on the calculated frequencies, thereby establishing the remarkable accuracy of conventional density-functional theory methods in determining the vibrational properties of adsorbed CO. Based on these results, we apply the electrochemical model to provide the first comprehensive ab-initio description of the vibrational Stark effect for CO on transition metal surfaces, finding excellent agreement with spectroscopic measurements. (cont.) As related projects, we have implemented a molecular-dynamics algorithm for metallic systems and developed a self-interaction correction method to rectify the tendency of density-functional theory calculations to overestimate binding energies. The present computational electrochemistry toolkit open promising perspectives for the application of first-principles methods to assist the microstructural engineering of electrochemical convertors.
Description
Thesis (Ph. D.)--Massachusetts Institute of Technology, Dept. of Materials Science and Engineering, 2008. Includes bibliographical references (p. 143-151).
Date issued
2008Department
Massachusetts Institute of Technology. Department of Materials Science and EngineeringPublisher
Massachusetts Institute of Technology
Keywords
Materials Science and Engineering.