Influence of Wake Model Superposition and Secondary Steering on Model-Based Wake Steering Control with SCADA Data Assimilation
Author(s)
Howland, Michael F.; Dabiri, John O.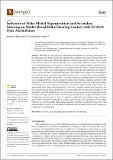
Downloadenergies-14-00052-v2.pdf (1.019Mb)
Publisher with Creative Commons License
Publisher with Creative Commons License
Creative Commons Attribution
Terms of use
Metadata
Show full item recordAbstract
Methods for wind farm power optimization through the use of wake steering often rely on engineering wake models due to the computational complexity associated with resolving wind farm dynamics numerically. Within the transient, turbulent atmospheric boundary layer, closed-loop control is required to dynamically adjust to evolving wind conditions, wherein the optimal wake model parameters are estimated as a function of time in a hybrid physics- and data-driven approach using supervisory control and data acquisition (SCADA) data. Analytic wake models rely on wake velocity deficit superposition methods to generalize the individual wake deficit to collective wind farm flow. In this study, the impact of the wake model superposition methodologies on closed-loop control are tested in large eddy simulations of the conventionally neutral atmospheric boundary layer with full Coriolis effects. A model for the non-vanishing lateral velocity trailing a yaw misaligned turbine, termed secondary steering, is also presented, validated, and tested in the closed-loop control framework. Modified linear and momentum conserving wake superposition methodologies increase the power production in closed-loop wake steering control statistically significantly more than linear superposition. While the secondary steering model increases the power production and reduces the predictive error associated with the wake model, the impact is not statistically significant. Modified linear and momentum conserving superposition using the proposed secondary steering model increase a six turbine array power production, compared to baseline control, in large eddy simulations by <inline-formula><math display="inline"><semantics><mrow><mn>7.5</mn><mo>%</mo></mrow></semantics></math></inline-formula> and <inline-formula><math display="inline"><semantics><mrow><mn>7.7</mn><mo>%</mo></mrow></semantics></math></inline-formula>, respectively, with wake model predictive mean absolute errors of <inline-formula><math display="inline"><semantics><mrow><mn>0.03</mn><mspace width="3.33333pt"></mspace><msub><mi>P</mi><mn>1</mn></msub></mrow></semantics></math></inline-formula> and <inline-formula><math display="inline"><semantics><mrow><mn>0.04</mn><mspace width="3.33333pt"></mspace><msub><mi>P</mi><mn>1</mn></msub></mrow></semantics></math></inline-formula>, respectively, where <inline-formula><math display="inline"><semantics><msub><mi>P</mi><mn>1</mn></msub></semantics></math></inline-formula> is the baseline power production of the leading turbine in the array. Ensemble Kalman filter parameter estimation significantly reduces the wake model predictive error for all wake deficit superposition and secondary steering cases compared to predefined model parameters.
Date issued
2020-12-24Department
Massachusetts Institute of Technology. Department of Civil and Environmental EngineeringPublisher
Multidisciplinary Digital Publishing Institute
Citation
Energies 14 (1): 52 (2021)
Version: Final published version